How animals follow their nose
It’s not easy to find the source of a swirling scent plume. Scientists are using experiments and simulations to uncover the varied strategies that animals employ.
Support sound science and smart stories
Help us make scientific knowledge accessible to all
Donate today
On October 2, 2022, four days after Hurricane Ian hit Florida, a search-and-rescue Rottweiler named Ares was walking the ravaged streets of Fort Myers when the moment came that he had been training for. Ares picked up a scent within a smashed home and raced upstairs, with his handler trailing behind, picking his way gingerly through the debris.
They found a man who had been trapped inside his bathroom for two days after the ceiling caved in. Some 152 people died in Ian, one of Florida’s worst hurricanes, but that lucky man survived thanks to Ares’ ability to follow a scent to its source.
We often take for granted the ability of a dog to find a person buried under rubble, a moth to follow a scent plume to its mate or a mosquito to smell the carbon dioxide you exhale. Yet navigating by nose is more difficult than it might appear, and scientists are still working out how animals do it.
“What makes it hard is that odors, unlike light and sound, don’t travel in a straight line,” says Gautam Reddy, a biological physicist at Harvard University who coauthored a survey of the way animals locate odor sources in the 2022 Annual Review of Condensed Matter Physics. You can see the problem by looking at a plume of cigarette smoke. At first it rises and travels in a more or less straight path, but very soon it starts to oscillate and finally it starts to tumble chaotically, in a process called turbulent flow. How could an animal follow such a convoluted route back to its origin?
Over the last couple of decades, a suite of new high-tech tools, ranging from genetic modification to virtual reality to mathematical models, have made it possible to explore olfactory navigation in radically different ways. The strategies that animals use, as well as their success rates, turn out to depend on a variety of factors, including the animal’s body shape, its cognitive abilities and the amount of turbulence in the odor plume. One day, this growing understanding may help scientists develop robots that can accomplish tasks that we now depend on animals for: dogs to search for missing people, pigs to search for truffles and, sometimes, rats to search for land mines.
The problem of tracking an odor seems as if it should have an elementary solution: Simply sniff around and head in the direction where the scent is strongest. Continue until you find the source.
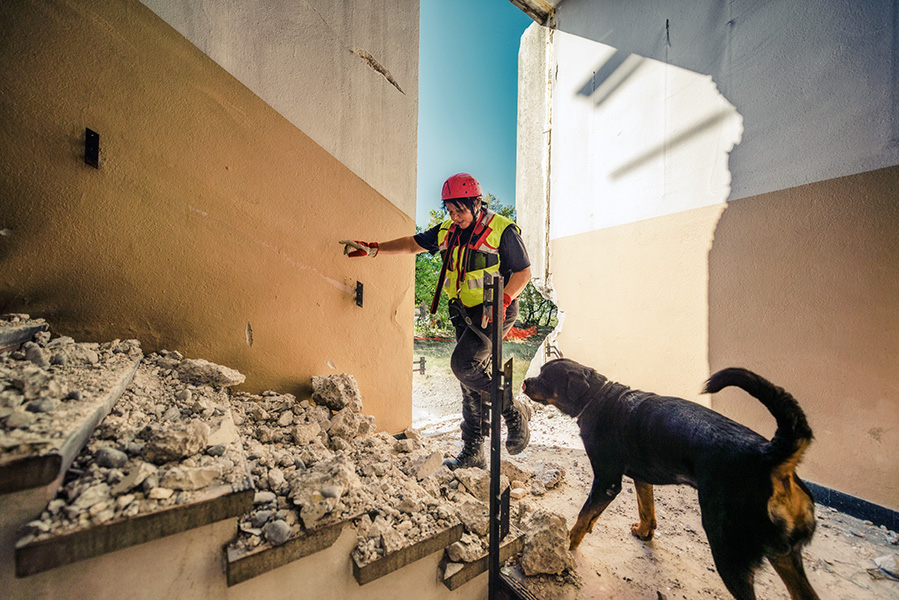
Search and rescue dogs rely on complex odor cues to find victims hidden under rubble. A combination of superior odor sensors and search strategies may be key to the animals’ abilities, which far exceed that of robotic devices.
CREDIT: ISTOCK.COM / EGON69
This strategy — called gradient search or chemotaxis — works quite well if the odor molecules are distributed in a well-mixed fog, which is the end stage of a process known as diffusion. But diffusion occurs very slowly, so thorough mixing can take a long time. In most natural situations, odors flow through the air in a narrow and sharply delineated stream, or plume. Such plumes, and the smells they convey, travel much more quickly than they would by diffusion. In some respects, this is good news for a predator, which can’t afford to wait hours to track its prey. But the news is not all good: Odor plumes are almost always turbulent, and turbulent flow makes searching by gradient wildly inefficient. At any given point, it’s quite possible that the direction in which the scent increases most rapidly could point away from the source.
Animals can call on a variety of other strategies. Flying insects, such as moths in search of a mate, adopt a “cast-and-surge” strategy, which is a form of anemotaxis, or response based on air currents. When a male moth detects a female’s pheromones, he will immediately start flying upwind, assuming there is a wind. If he loses the scent — which probably will happen, especially when he is far away from the female — he will then start “casting” from side to side in the wind. When he finds the plume again, he will resume flying upwind (the “surge”) and repeat this behavior until he sees the female.
Some land-bound insects may use a strategy called tropotaxis, which could be thought of as smelling in stereo: Compare the strength of the smell at the two antennae and turn toward the antenna getting the strongest signal. Mammals, which typically have nostrils that are more narrowly spaced relative to body size than an insect’s antennae, often use a comparison-shopping strategy called klinotaxis: Turn your head and sniff on one side, turn your head and sniff on the other side, and turn your body in the direction of the stronger smell. This requires a slightly higher level of cognition because of the need to retain a memory of the most recent sniff.
The odor environment may dictate the best search strategy for finding the source of an odor. If odor molecules diffuse evenly from the source (top), it will be at the center of the cloud so an animal can simply head toward the highest concentration of odor. If the odor plume is turbulent (middle), the concentration strategy can still work if the animal is close enough to the source, despite the odorant’s uneven concentration. If the animal is far from the source (bottom), the odor plume breaks up into discrete “packets,” so the animal only catches the scent intermittently. In this case, the animal needs a more complex search strategy in which it casts about whenever it loses the scent.
Odor-sensing robots may have another strategy they can draw on — one that nature might never have come up with. In 2007, physicist Massimo Vergassola of l’École Normale Supérieure in Paris, proposed a strategy called infotaxis, in which olfaction meets the information age. While most of the other strategies are purely reactive, in infotaxis the navigator creates a mental model of where the source is likeliest to be, given the information it has previously collected. It will then move in the direction that maximizes information about the source of the smell.
The robot will either move toward the most likely direction of the source (exploiting its previous knowledge) or toward the direction about which it has the least information (exploring for more information). Its goal is to find the combination of exploitation and exploration that maximizes the expected gain in information. In the early stages, exploration is better; as the navigator gets closer to the source, exploitation is the better bet. In simulations, navigators using this strategy travel paths that look a lot like the cast-and-surge trajectories of moths.
In Vergassola’s earliest version, the navigator needs to make a mental map of its surroundings and calculate a mathematical quantity called Shannon entropy, a measure of unpredictability that is high in directions the navigator has not explored and low in directions it has explored. This probably requires cognitive abilities that animals do not possess. But Vergassola and others have developed newer versions of infotaxis that are less computationally demanding. An animal, for example, “can take short cuts, maybe approximate the solution to within 20 percent, which is pretty good,” says Vergassola, a coauthor of the Annual Reviews article.
Infotaxis, klinotaxis, tropotaxis, anemotaxis … which taxis will get you to your destination first? One way to figure that out is to go beyond qualitative observations of animal behavior and to program a virtual critter. Researchers can then figure out the success rate of various strategies under a variety of situations in both air and water. “We can manipulate far more things,” says Bard Ermentrout, a mathematician at the University of Pittsburgh and a member of Odor2Action, a 72-person research group organized by John Crimaldi, a fluid dynamicist at the University of Colorado, Boulder. For example, researchers can test how well a fly’s strategy would work underwater, or they can ramp up the turbulence of the fluid and see when a particular search strategy starts to fail.
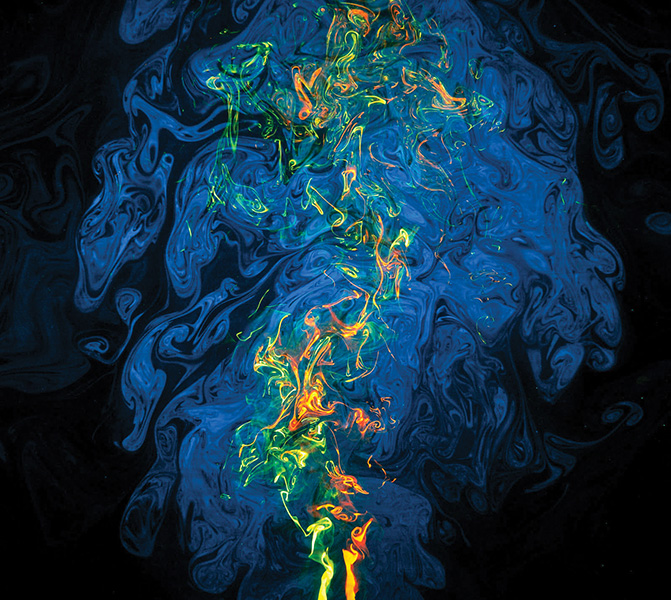
Turbulence makes scent plumes hard to follow. Here, two dyes — one green, one red — are injected into a turbulent stream of water (bottom). The turbulence breaks up the streams into fragments. Imagine starting at the top of the image and trying to follow the colors to their source.
CREDIT: M. KREE ET AL / PHYSICS OF FLUIDS 2013
So far, simulations show that when turbulence is low, both stereo smelling and comparison shopping work most of the time — though, as expected, the former works better for animals with widely spaced sensors (think insects) and the latter works better for animals with closely spaced sensors (think mammals). For high turbulence, though, a simulated animal doesn’t perform well with either approach. Yet real mice hardly seem fazed by a turbulent plume, lab experiments show. This suggests that mice may still have tricks we don’t know about, or that our description of klinotaxis is too simple.
Furthermore, while simulations can tell you what an animal might do, they don’t necessarily say what it does. And we still don’t have a way to ask the animal, “What is your strategy?” But high-tech experiments with fruit flies are getting closer and closer to that Dr. Dolittle-style dream.
Fruit flies are in many ways ideal organisms for smell research. Their olfactory systems are simple, with only about 50 kinds of receptors (compared to about 400 in humans, and more than 1,000 in mice). Their brains are also relatively simple, and the connections between neurons in their central brain have been mapped: The fruit fly’s connectome, a sort of wiring diagram for its central brain, was published in 2020. “You can look up any neuron and see whom it’s connected to,” says Katherine Nagel, a neuroscientist at New York University and another of the Odor2Action team members. Before, the brain was a black box; now researchers like Nagel can just look the connections up.
One of the puzzles about flies is that they appear to use a different version of the “surge-and-cast” strategy than moths. “We noticed that flies, when they encounter an odor plume, would usually turn toward the center line of the plume,” says Thierry Emonet, a biophysicist at Yale University. Once they find the center line, the source is most likely to be directly upwind. “[We] asked, how the heck does the fly know where the center of the plume is?”
Emonet and his collaborator Damon Clark (a physicist whose lab is next door) have answered this question with an ingenious combination of virtual reality and genetically modified flies. In the early 2000s, researchers developed mutant flies with olfactory neurons that respond to light. “It turns the antenna into a primitive eye, so we can study olfaction the way that we study vision,” says Clark.
This solved one of the biggest problems in smell research: You usually can’t see the odor plume that an animal is responding to. Now you can not only see it, you can project a movie of any odor landscape you want. The genetically modified fly will perceive this virtual reality as a smell and respond to it accordingly. Another mutation rendered the flies blind, so that their actual vision wouldn’t interfere with the visual “odor.”
In their experiments, Clark and Emonet put these genetically modified flies in a container that confines their motion to two dimensions. After the flies got accustomed to the arena, the researchers presented them with a visual odor landscape consisting of moving stripes. The flies always walked toward the oncoming stripes, they found.
Next, Clark and Emonet presented a more realistic odor landscape, with turbulent twists and swirls copied from real plumes. The flies were able to navigate successfully to the center of the plume. Finally, the researchers projected a time-reversed movie of the very same plume, so that the average motion of the odor in the virtual plume was toward the center, rather than away — an experiment that could not possibly be done with a real odor plume. The flies were confused by this bizarro-world plume and moved away from the center rather than toward it.
This video shows how flies navigate by smelling how odors are moving. The first part of the video shows computer simulations of flies programmed to turn so that the odors are coming directly toward them; over time, this leads the flies to move toward the source, even in a turbulent scent plume. After the 25 second mark, the video shows actual flies, genetically modified so that their scent-detecting cells respond to light, following a video “scent” plume projected on the floor of their container. The flies respond in much the same way as the simulated flies.
CREDIT: YALECAMPUS
Flies, Clark and Emonet concluded, must sense the motion of odor packets, as Emonet calls discrete clumps of odor molecules. Think about this for a second: When you smell the neighbor’s barbecue, can you tell whether the smoke particles passing your nose are traveling from left to right, or right to left? It’s not obvious. But a fly can tell — and olfaction researchers have previously overlooked this possibility.
How does sensing the motion of odor molecules help the fly find the center of the plume? The key point is that at any given time, there are more odor molecules traveling away from the center of the plume than toward it. As Emonet explains, “the number of packets in the center line is higher than away from it. So you get a lot of packets in the center moving away, and not as many from the outside moving in. Each packet individually has equal probability of moving in any direction, but collectively there is a dispersion away from the center.”
In fact, the flies are processing the incoming sensory information in a remarkably sophisticated way. In a windy environment, the direction the fly travels is actually a combination of two distinct directions, the direction of air flow and the average direction the odor packets are moving. By using the fly connectome, Nagel has pinpointed one of the places in the brain where this processing must occur. The fly’s wind-sensing neurons crisscross over its olfactory direction-sensing neurons at a particular place in the brain that’s descriptively called the “fan-shaped body.” Together, the two sets of neurons tell the fly which direction to move in.
In other words, the fly is not just reacting to its sensory inputs but also combining them. Since each set of directions is what mathematicians call a vector, the combination is a vector sum. It’s possible, says Nagel, that the flies are literally adding vectors. If so, their neurons are performing a calculation that human college students learn how to do in vector calculus.
Flies can tell where the center of an odor plume lies by monitoring which direction most odor molecules are moving, since more molecules move away from the center of the plume than toward it. A part of the fly’s brain called the fan-shaped body combines this odor information (carried by a group of nerve cells called tangential neurons) with information on wind direction (encoded by different neurons called columnar neurons), to determine the locations of the scent’s source.
Nagel plans to look next for similar neural structures in the brains of crustaceans. “The odor is completely different, the locomotion is different, but this central complex region is conserved,” she says. “Are they doing fundamentally the same thing as flies?”
While the connectome and virtual-reality experiments are producing amazing insights, there are many questions left to be answered. How do dogs like Ares track a smell that is partly on the ground and partly in the air? How do they allocate their time between sniffing the ground and sniffing the air? For that matter, how does “sniffing” work? Many animals actively disturb the airflow, rather than just passively receiving it; mice, for example, “whisk” with their whiskers. How do they use this information?
And what other non-human abilities might animals possess, akin to the flies’ ability to detect the motion of an odor packet? These and many more mysteries are likely to keep biologists, physicists and mathematicians sniffing for answers for a long time.
10.1146/knowable-030623-4
TAKE A DEEPER DIVE | Explore Related Scholarly Articles