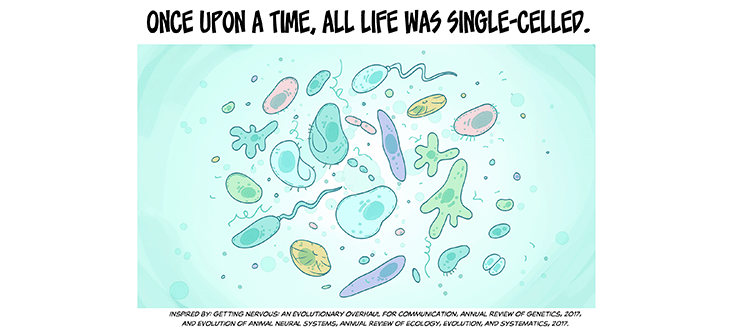
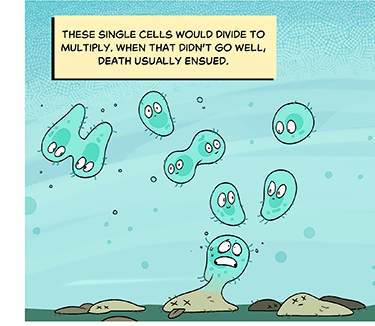
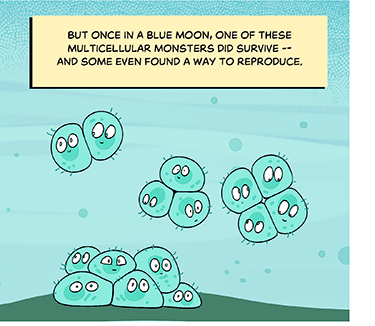

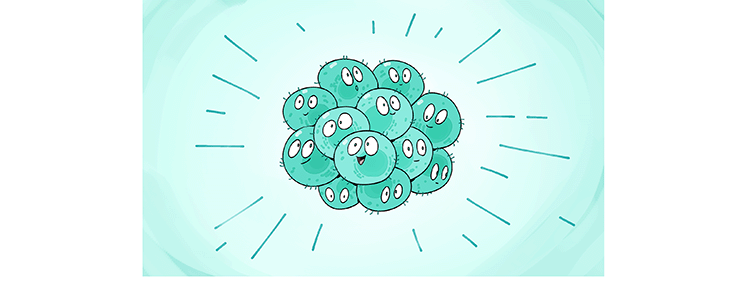
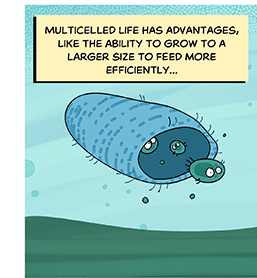
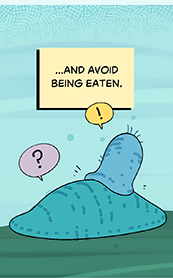
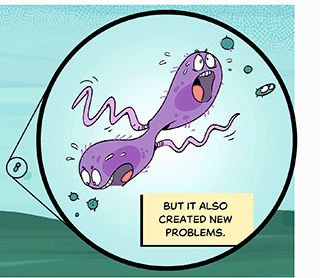
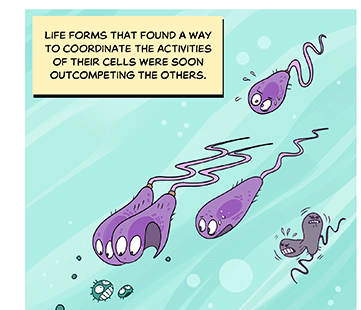
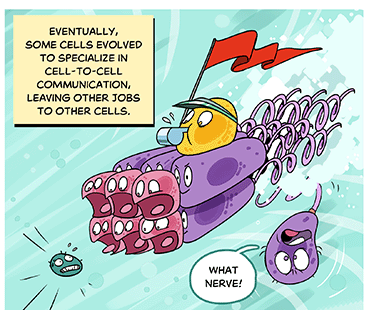

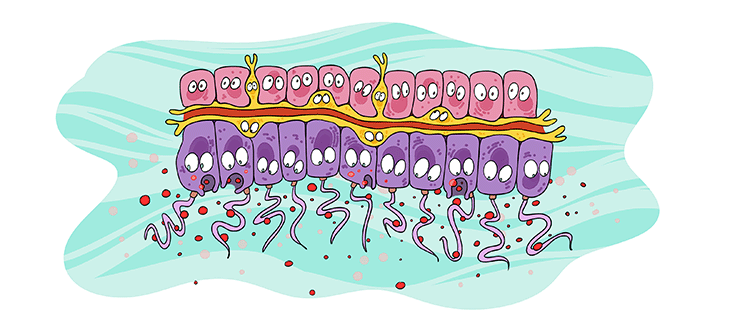

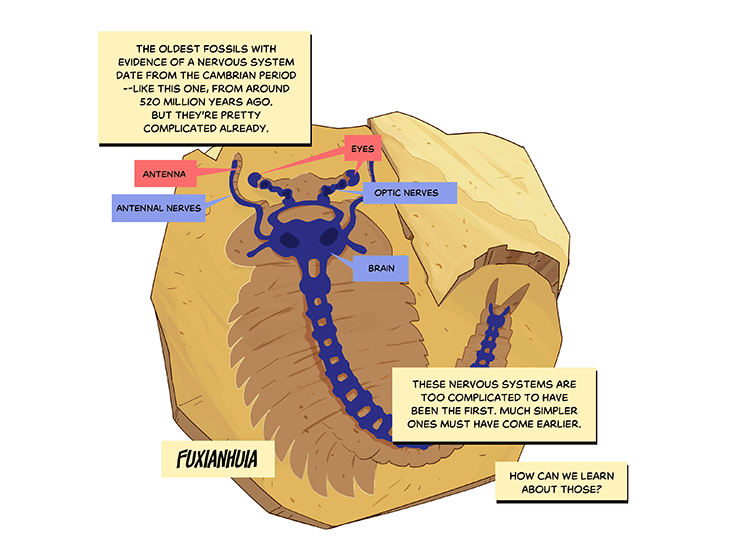
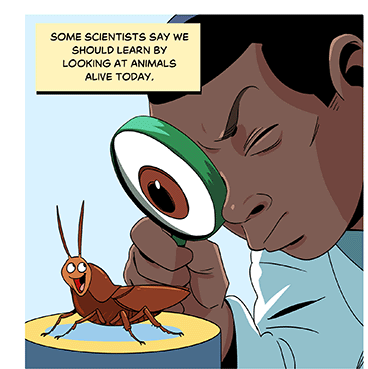
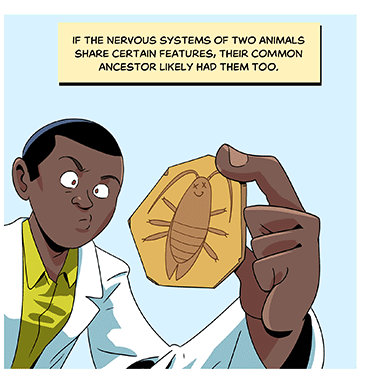
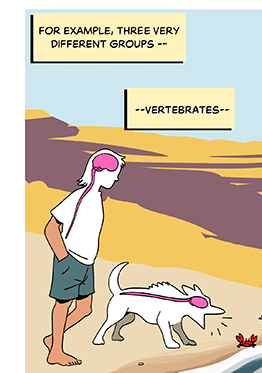
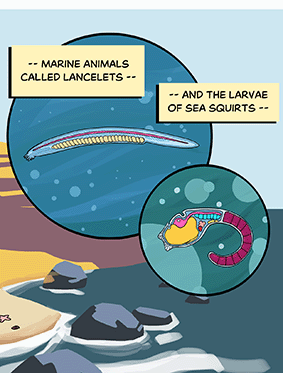
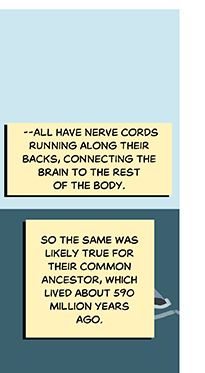
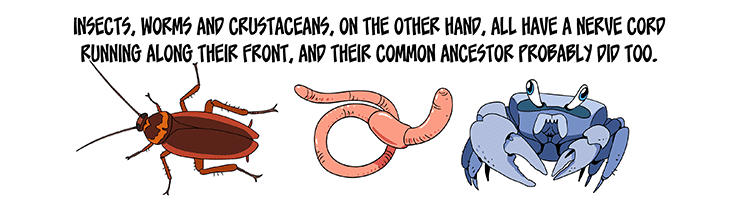
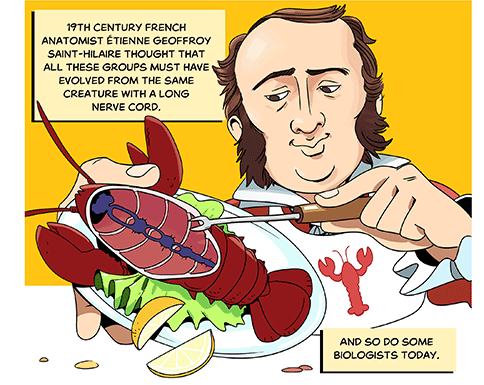
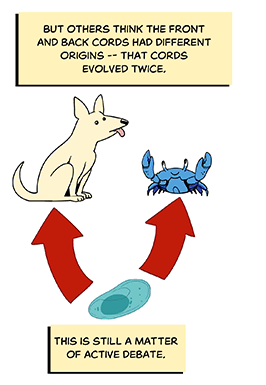
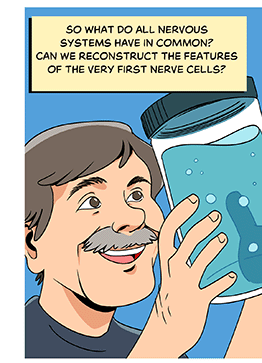
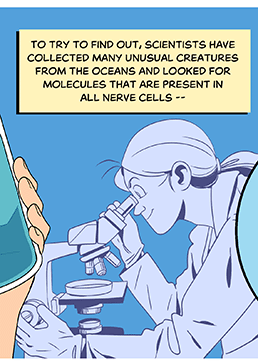
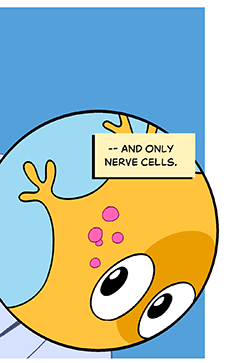
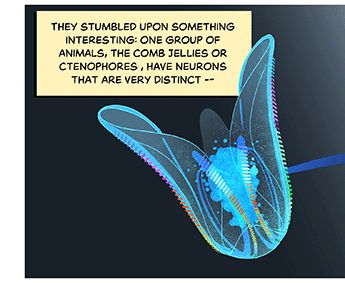
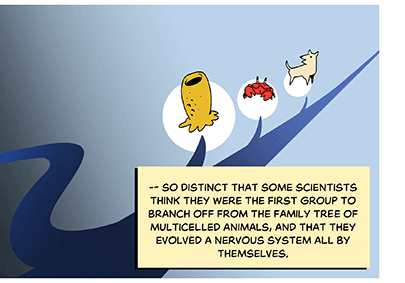
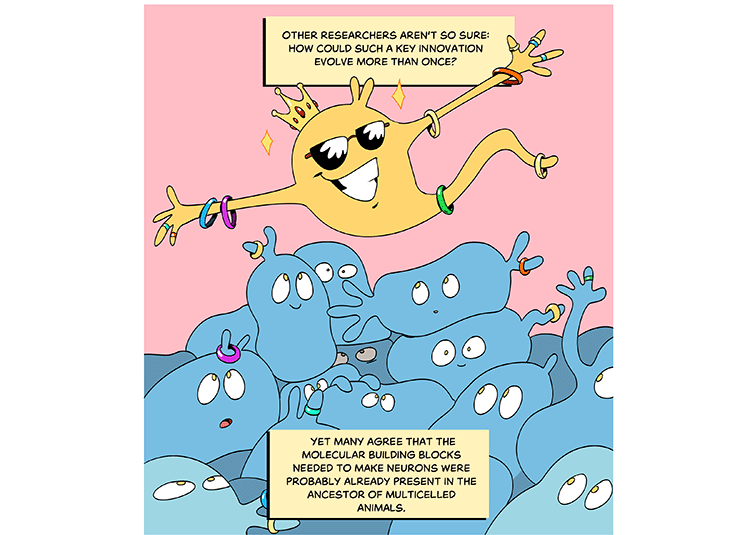
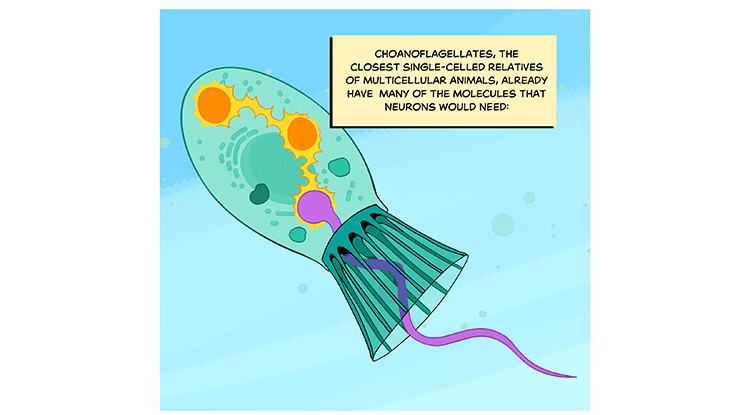
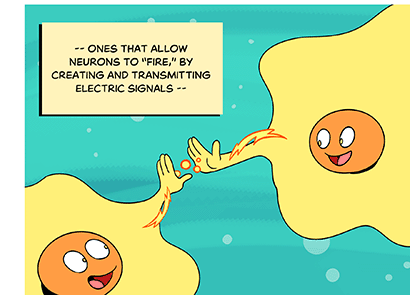
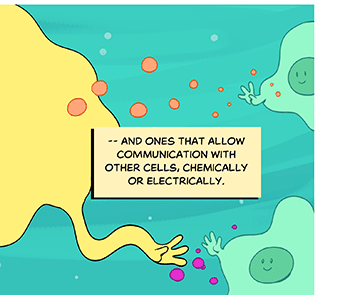

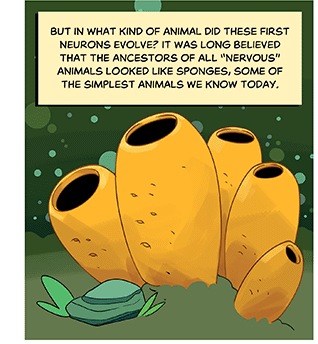
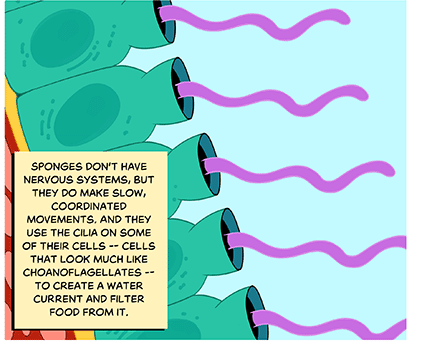
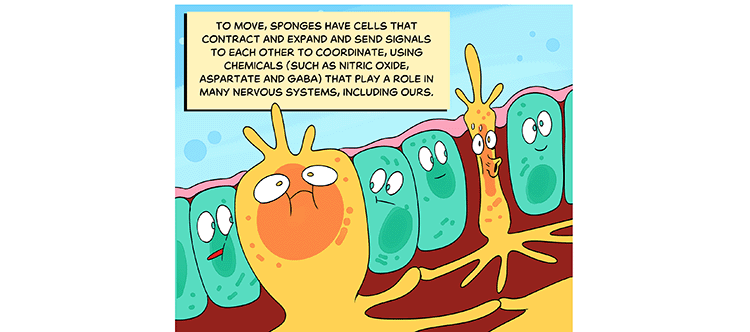
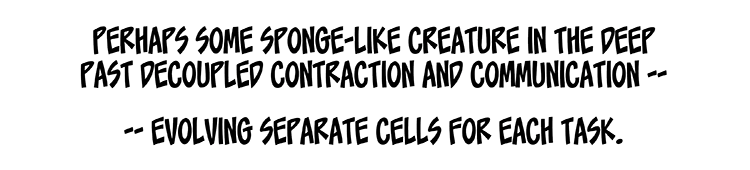
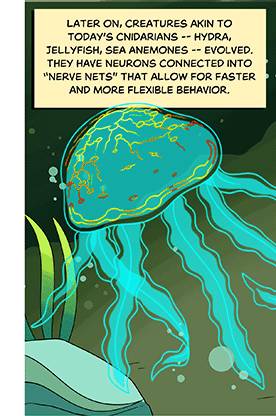
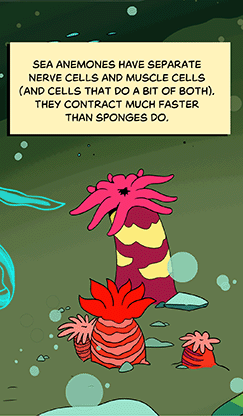
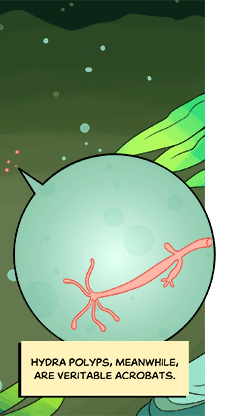
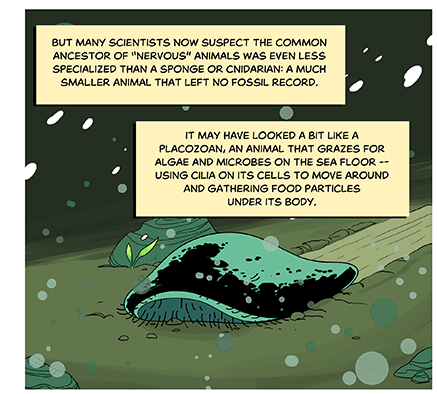
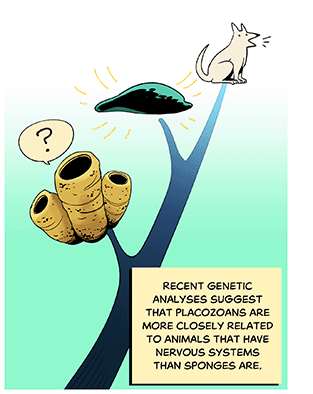
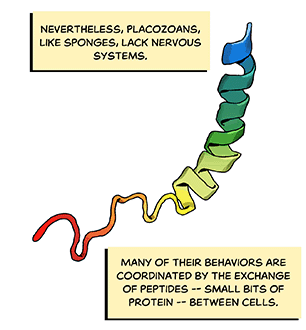
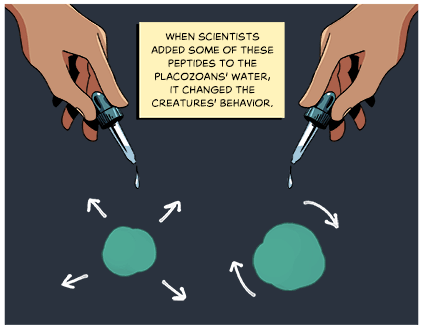
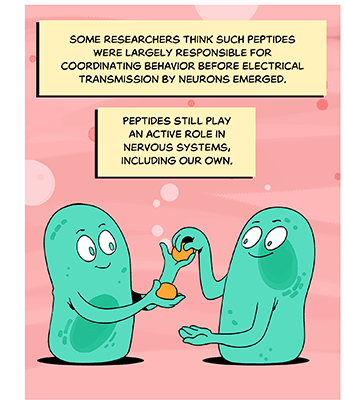
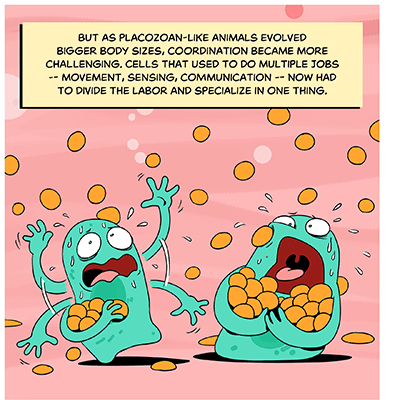
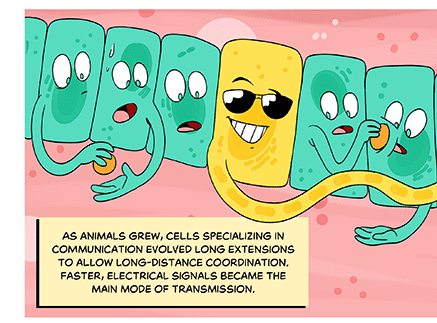
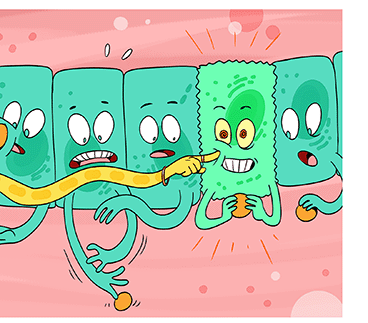
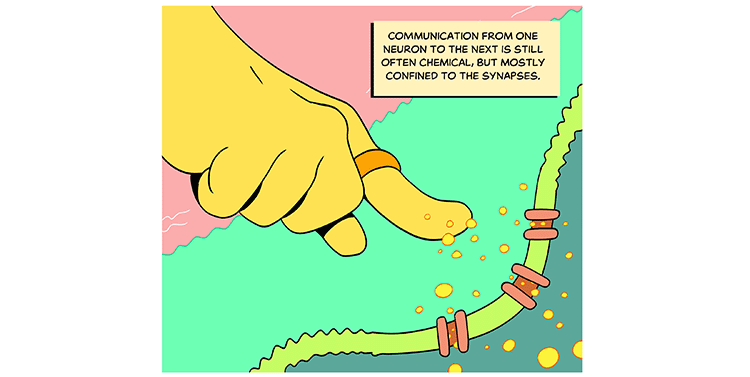
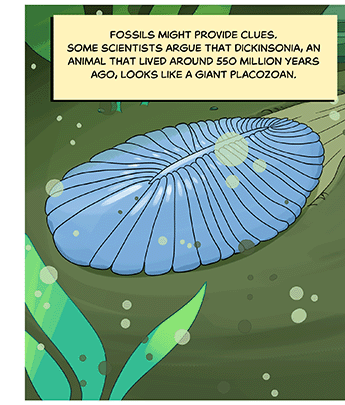
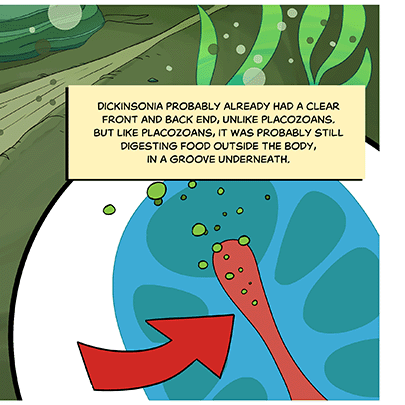
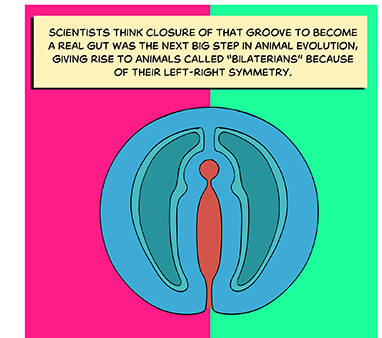
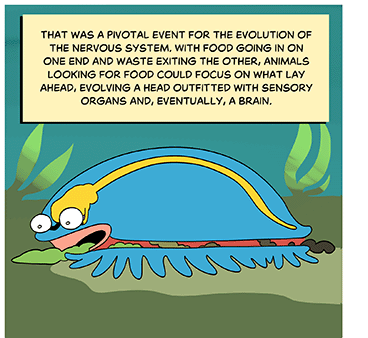

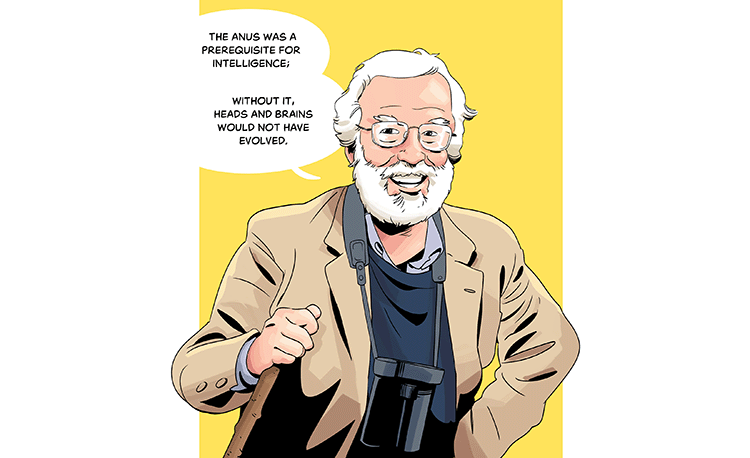
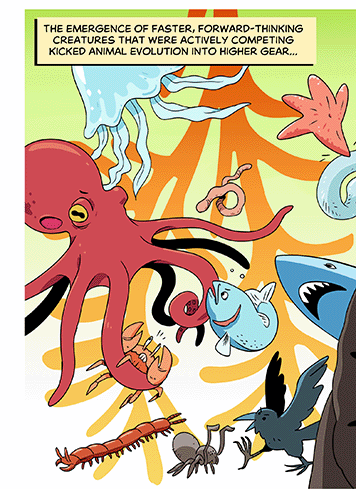
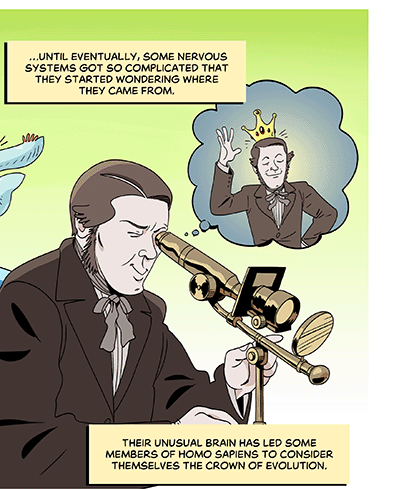

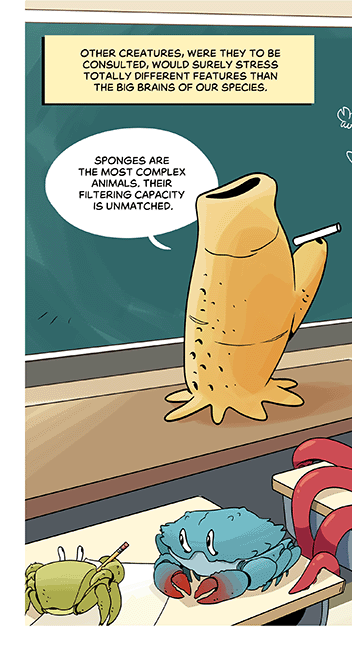
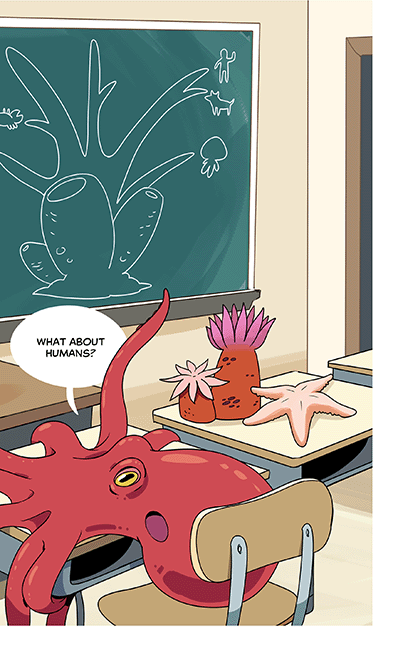
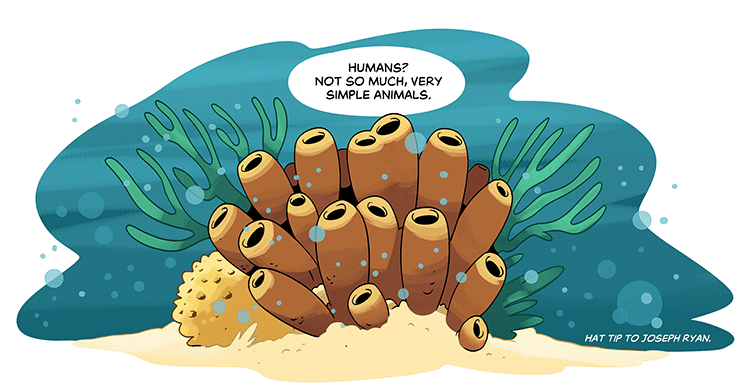
COMIC: When, why and how did neurons first evolve? Scientists are piecing together the ancient story.
Support sound science and smart stories
Help us make scientific knowledge accessible to all
Donate today
10.1146/knowable-091323-1
Maki Naro is a feral cartoonist and science communicator. You can find his work at makinaro.com.
TAKE A DEEPER DIVE | Explore Related Scholarly Articles
Thank you for your interest in republishing! This HTML is pre-formatted to adhere to our guidelines, which include: Crediting both the author and Knowable Magazine; preserving all hyperlinks; including the canonical link to the original article in the article metadata. Article text (including the headline) may not be edited without prior permission from Knowable Magazine staff. Photographs and illustrations are not included in this license. Please see our full guidelines for more information.