Imagine a tennis ball partially covered with fuzz that reacts chemically with salt. Plop the ball into the ocean and the reaction drives the ball through the water as molecules bombard it. They won’t push the tennis ball very far. But shrink the ball to the nanoscale, and the tiny sphere becomes a swimmer with no motor.
Such machine-free movement is called phoretic self-propulsion, and it’s not imaginary. Early experiments show that it holds promise for targeted drug delivery and environmental monitoring and remediation. But there’s still some basic physics left to be explored.
“This is very space-age thought,” says Jonathan Posner, an engineer at the University of Washington in Seattle. Self-propelled particles are “tremendously simple,” he says.
Start with a solid sphere, half of which is coated with a chemically reactive surface, say platinum. When put in a fluid, such as hydrogen peroxide, the platinum and peroxide interact, changing the concentrations of the chemicals surrounding one side of the sphere. The reactive half gathers what’s produced by the platinum-peroxide reaction on its surface, while the other half remains unchanged. The difference creates a chemical gradient in the fluid along which the sphere flows, so the sphere “swims” forward.
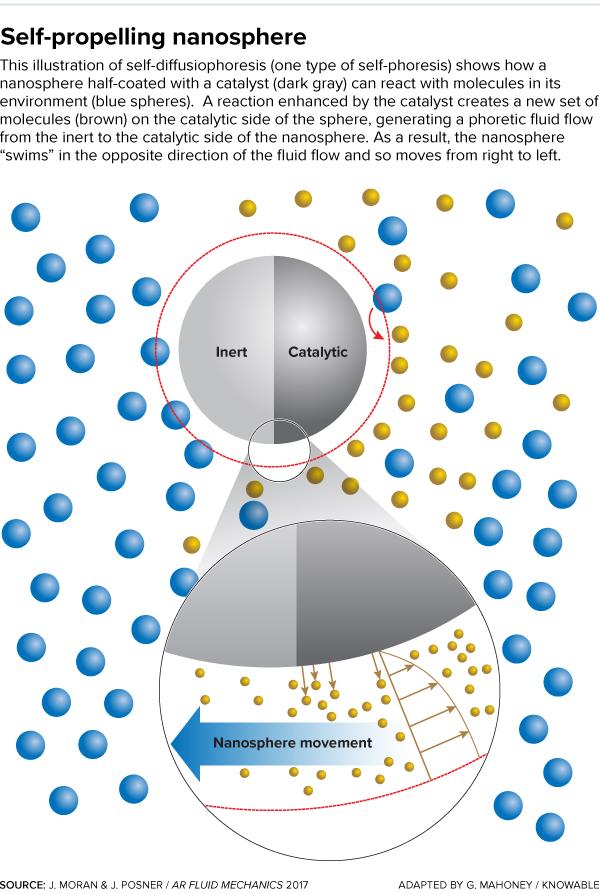
Posner and Jeffrey Moran of MIT describe the physics behind phoretic self-propulsion in the 2017 Annual Review of Fluid Mechanics.
“So what do we do with this cool science?” Posner says. “Something useful.” Scientists imagine one day injecting these particles into the bloodstream and watching them move toward and then glom onto cancer cells to deliver a drug that kills them. Or there’s an expanding oil spill. Self-phoretic particles could be engineered to move toward the oil, interact with it and turn it into less noxious components, such as carbon dioxide and water.
“You are starting to see a glimpse of this,” Posner says, describing a 2015 paper by researchers who had mice swallow zinc-coated particles. The particles drove themselves into the animals’ stomach walls, where they deposited therapeutic gold nanoparticles. Posner and Moran also note a 2016 paper describing how iron-propelled particles moved toward a dye and then degraded it.
Such experiments show the potential of phoretic self-propulsion, and the tennis ball analogy offers an accessible way to think about it. But, despite 40 years of efforts to understand the phenomenon, working out the physical details of the various types of phoretic self-propulsion has proved difficult. The scientific community continues to disagree on many of the issues, says applied mathematician Eric Lauga of Cambridge University.
Posner says there is still much to work out about phoretic self-propulsion, noting that the particles can be finicky. If the properties of the surrounding liquid are tweaked just a bit — if, say, the salt content is higher than normal — then the particles move more slowly, he explains. This can also happen in thick biological liquids, such as mucus and blood, making the prospect of drug delivery even more challenging.
Yet scientists studying self-phoresis are optimistic. “A lot of creative work is done in experimental labs, often by engineers and applied physicists, who are way ahead of theorists,” Lauga says. That’s “leading to a wide variety of new phenomena we can only begin to understand.”