Light is an adept acrobat. It bounces off mirrors, bends through lenses to magnify matter and carries messages through fibers. But light can’t sidestep objects in its path — avoiding them would require light to bend backward. Light needs help to do that.
For decades, researchers have been working to build materials that can manipulate light in just that way. Similar materials can camouflage sound. Such metamaterials could endow light and sound waves with many new talents. Perhaps the most tantalizing work has been contouring the two to create invisibility cloaks, potentially enabling an object to disappear from sight or detection by sound. Such invisibility cloaks ignite the imagination, conjuring up comparisons to magical wizard Harry Potter’s cloak or the Romulan cloaking device in Star Trek.
But long before such cloaking technology appears under the Christmas tree, research on metamaterials and related technology could profoundly affect sound recording and soundproofing, photography and microscopy, and Internet communications. Researchers are working on materials to stop sound in its tracks, to magnify matter with ever-thinner lenses, and to manipulate the movement of radio waves and microwaves for Wi-Fi. The research even has the potential to rein in the seismic waves of earthquakes.
“Metamaterials give us a way to control waves in ways we’ve never had before,” says Duke University engineer David Smith.
Going quiet
Metamaterials first gained attention for their incredible ability to manipulate light. Typically, they are designed from tiny elements of metal or plastic, which are arranged into repeating patterns smaller than the wavelengths of light or sound that researchers want to manipulate. The precise arrangement determines the material’s refractive index — a quantitative figure describing how much it refracts, or bends, an electromagnetic wave. Researchers can design metamaterials with a negative refractive index, meaning the material bends light backwards. It would be like sticking a spoon in water and seeing the round end stick straight back out. New metamaterials that do something similar with sound waves are now gaining momentum. And they might help make the world a quieter place.
Total sound absorption is one goal of metamaterial research that holds great promise for commercial applications, Min Yang and Ping Sheng of Hong Kong University of Science and Technology wrote last year in the Annual Review of Materials Research. The possibilities are expansive, Sheng says.
Noise is everywhere. Machines, planes, trains, cars, cooling fans for computers — just about everything gives off some sort of sound. Some normal materials, such as spongy soundproofing textiles, can absorb and reflect sound, but often without allowing ventilation. That means such materials aren’t ideal for soundproofing computer servers, fans, air-conditioning systems and recording studios, examples where air needs to flow so heat can be transferred and dissipated.
Engineer Yun Jing and his colleagues at North Carolina State University have come up with one design to deal with the problem. To keep these systems soundproof yet ventilated, the researchers have been working on what they call an acoustic “metacage,” a circular ring of CD-case-shaped square structures with tiny gaps, or open channels, in between. A noisemaker, such as a stereo speaker or a fan, sits at the center of the ring. The metacage manipulates the sound waves from the speaker so they can’t escape through the gaps, but air flowing through the structure can move freely.
A conventional material serving as a sound barrier (left) does not permit the ventilation needed to cool the noise-producing fan. A metacage consisting of special resonators and gaps to allow airflow can eliminate escaping noise while still providing ventilation. Such cages could be useful in soundproofing computer servers or possibly recording studios.
CREDIT: C. SHEN ET AL / JOURNAL OF APPLIED PHYSICS 2018
The technology relies on Helmholtz resonance, a phenomenon most know well without knowing its name. If you’ve ever blown air across the top of an empty bottle so it hums, you’ve created it. The metamaterials forming Jing’s metacage are composed of open channels and Helmholtz resonators sensitive to specific frequencies of sound. When sound waves hit the walls of the metacage, the waves are trapped, Jing and colleagues reported in August 2017 in a paper on arXiv.org.
Seismic waves emitted during earthquakes behave similarly to sound waves, so the development of devices to dampen sound might one day help shield vulnerable structures from shaking. Metamaterials would block the incoming seismic waves by interfering with them.
Focusing light
Aside from the potential to mellow the effects of seismic waves, metamaterials are also now poised to change the way we picture the world. Inside a cell phone, for example, is a camera made of layers of glass — each layer is a refractive lens. When each lens is perfectly aligned with the lens on top of it and all the others, the camera produces crisp images, capturing spectacular colors. Even with the latest, slimmest phones, those lenses still make the devices breakable and bulky, with a little bump sticking out where the phone’s camera is positioned.
“Companies are extremely interested in eliminating that bump,” says Federico Capasso, a physicist at Harvard University. With metamaterials, he and colleagues have made a single-layer lens that could cut phone cameras’ size by a third or a half. Similar size reductions are possible for security cameras and for cameras in cars and trucks.
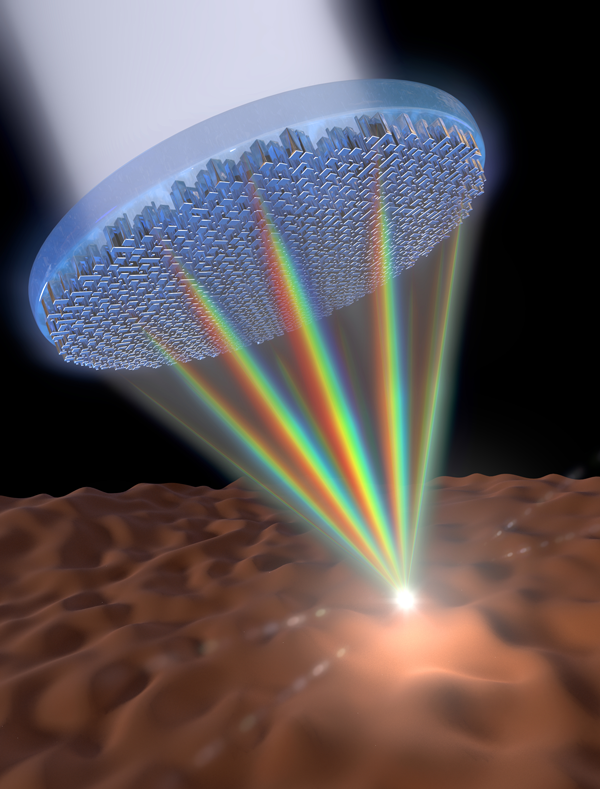
A flat metalens built from titanium dioxide “nanofins” can focus all the colors of visible light in the same spot with high resolution and could allow the lenses to be used in commercial applications such as cameras.
CREDIT: COURTESY OF JARED SISLER / HARVARD SEAS
Capasso and his colleagues designed the flat lens using arrays of tiny titanium dioxide towers. Each tower stands only 600 nanometers tall. When arranged in specific patterns, the towers together can manipulate the direction the light travels so that its different colors all reach the same focal point at exactly the same time. This is fairly easy to do with a single color, or wavelength, of light, but it’s much more challenging when trying to focus the wavelengths of red and blue light and all the colors in between.
Capasso explains that in air, light travels at a constant 300,000 kilometers per second. But when waves of light hit a material, their speed changes, depending on wavelength. Red wavelengths are longer, so when they hit a tower, more of the light remains in the air, compared with blue wavelengths, which are shorter and interact more directly with a tower. Knowing this, the researchers developed paired towers that could manipulate the speed of different wavelengths of light simultaneously, creating time delays so that all of the wavelengths of light reach the focal point of the lens at the same time.
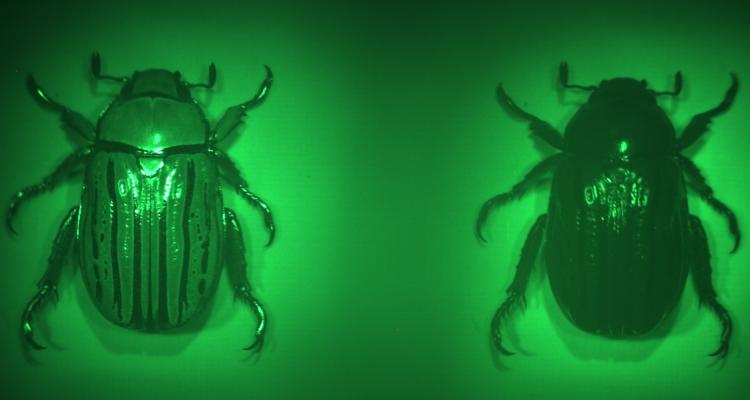
Two images of a beetle (Chrysina gloriosa), illuminated by green LED light, were formed by a chiral metalens in the field of view of a color camera. Such an image shows how the lens could be used to study chiral molecules of different shapes and sizes without additional optical equipment.
CREDIT: CAPASSO LAB / HARVARDSEAS
“It’s a very nifty and nontrivial trick,” Capasso says of his current lens, which is thinner than a human hair. His team described the latest lens in January in Nature Nanotechnology. And in November 2017, the researchers posted a paper on arXiv.org describing a metalens just a bit bigger and maybe more useful — millimeters to centimeters in diameter and less than a micrometer thick. It uses silicon towers to manipulate light and can be fabricated in the same way as microchips, so it can be mass-produced, perhaps much more easily than with the grinding and polishing needed to make traditional glass lenses. Ultimately, Capasso says, the team would like to make these lenses with a diameter of about a centimeter, which would open possibilities for the metalenses to be used in virtual reality and other tech devices.
“I have quite critical views about the traditional metamaterial work. What is the technological impact?” Capasso says. So far, most metamaterials research has not made it out of the lab. But he thinks the metalens is attainable in the near future. He and his colleagues are negotiating with cell phone companies and other tech innovators to transition from glass to metamaterial lenses.
Broadening connections
Beyond better sound systems and cameras, metamaterials are on the verge of changing how we communicate. “There is an unending thirst for connectivity on a global scale, and it’s been growing exponentially for the last 20 years, with no end in sight,” says Nathan Kundtz, president of Kymeta, a company producing metamaterial-based antennas.
Cellular networks help us manage that thirst for connectivity, providing data when we’re mobile. But those networks have limitations, including spotty coverage and slower data rates compared with fiber-optic cables or wired telephone lines. And the bandwidth at cellular frequencies is filling up fast. That’s why he has proposed tapping into higher frequencies, those in the microwave realm of the electromagnetic spectrum, specifically what’s called the Ka-band at 27 to 40 gigahertz. Cellular frequencies are typically in the megahertz range.
“If you go into the frequencies that are used for high-capacity satellites, there’s literally 5,000 times more spectrum available than there is available in the cellular band,” Kundtz says. “What that means is that you have a tremendous amount of capacity that is currently untapped, or largely untapped.”
Tapping that spectrum requires satellites in orbit and antennas that can send the signal a long way. Traditional antennas are like those big hunks of metal installed by satellite TV companies. There’s tremendous capacity there, but it’s not really mobile, even though it’s wireless. “That's one of the reasons we’ve historically seen satellites be underadopted and underdeveloped,” Kundtz says.
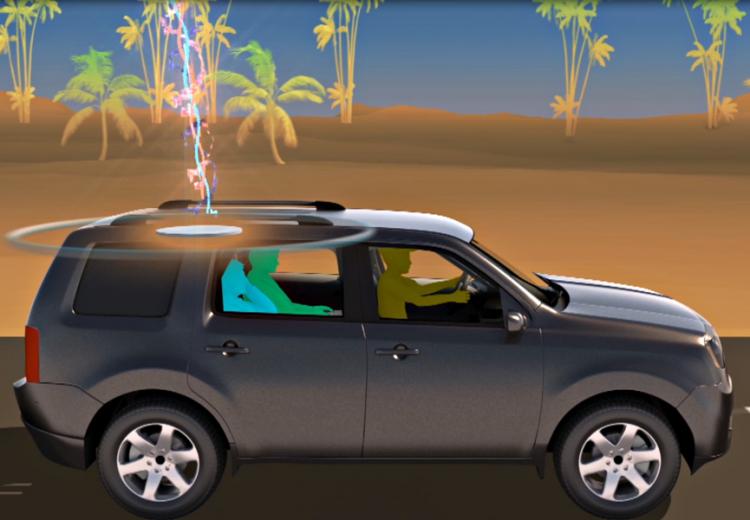
Arranging naturally occurring materials in a specific pattern generates a metamaterial that forms holographic beams (shown) capable of linking to satellites.
CREDIT: KYMETA
Just after he finished his PhD with David Smith at Duke University, Kundtz moved to the West Coast to see if he could connect what he knew about metamaterials with what was needed to make mobile antennas. His solution: a satellite dish that doesn’t physically adjust to find a signal. Instead the flat panel “positions” itself electronically to tune into a signal, with no major moving parts. The antenna, he says, is covered in a holographic surface made of 30,000 metamaterial elements, which act as tiny resonators that can be turned on and off using liquid crystals. The resonators ring loudly at specific frequencies and scatter energy out of the antenna when they’re turned on but not when they’re off. They form beams that can connect and communicate with satellites even when the entire antenna is in motion — on the back of a car, for example, Kundtz says.
In essence, the resonators function exactly like pixels on a TV. Because of the similarities, the antennas can even be manufactured on production lines for LCD television screens. Kundtz and colleagues describe the details of the antennas in a paper posted at arXiv.org in November 2017.
While his explanation of the antennas seems breezy now, building them was extremely hard. “Launching rockets has nothing on how difficult it is to make an electronically steered antenna, I’ll tell you that,” Kundtz says. Still, despite the difficulty, the hexagonal dishes have been manufactured and are being installed on yachts and cars and could even be installed on aircraft to provide Internet to passengers. All it takes is a satellite connection and a pizza-size antenna that can see the sky.
“What will that touch?” Kundtz asks. “I think just about everything.”
Capasso says it’s a fair bet that Kundtz and his colleagues are onto something marketable with the metamaterial antennas. They, along with soundproof cages and superthin lenses, obviously aren’t as flashy as invisibility cloaks. But invisibility is far from being practical, Capasso says. “If you want to hide something, you have got to be able to hide it over a very wide frequency range,” essentially covering all wavelengths of light, he says. “It’s very difficult.”