In a dimly lit basement at the University of Illinois at Urbana-Champaign, not far from a nurtured, sunlit plot of corn at the center of campus, there’s a torture chamber for plants. It looks pretty innocuous: a plywood box the size of a small coffee table, with air sifting in through a tube and out from a fist-size hole in the lid. The box has two tiers. The top holds tiny, nine-day-old tobacco seedlings, no bigger than your thumbnail. The bottom is layered with soda lime, a granular, Kitty-Litter-like material. It’s the same stuff they use in submarines to scrub carbon dioxide from the air.
For someone in a submarine, getting rid of carbon dioxide is life-saving. For the little plants in the box, it is a death sentence — unless, that is, they’ve inherited a protective genetic tweak. If a seedling can sweat it out for 24 hours and emerge with its chloroplasts unruffled, the UI scientists know they’ve got something special.
Plants need just three basic ingredients — sun, water and carbon dioxide in the air — to create the sugars that ultimately sustain most of life on Earth. Photosynthesis makes the world go round: It provides the oxygen we breathe, the food we eat and the fuel we burn. But though it is a marvel, it is also stupefyingly inefficient. From a given amount of sunshine, most plants convert less than five percent of that light energy into biomass, and under some environmental conditions, as little as one percent.
And yet that very inefficiency is giving scientists hope, because it offers room for improvement and a way to provide for humankind’s future. If plants could photosynthesize better, the extra growth might help feed the nearly 10 billion people that the United Nations estimates will populate the planet by 2050. And it could help offset other anticipated challenges: global dietary shifts toward meat and dairy, an uptick in demand for biofuels that leaves less land available to grow food, and a hotter landscape that will meddle with many crops’ photosynthesizing skills. “There is much uncertainty about how we will get to the yield increases we need in the future,” says agricultural scientist Tony Fischer of the Commonwealth Scientific and Industrial Research Organization in Australia. “We need to be trying every tool in the toolbox.”
Part of the solution may lie with a little seedling gasping for air, right now, in the box in that Illinois basement.
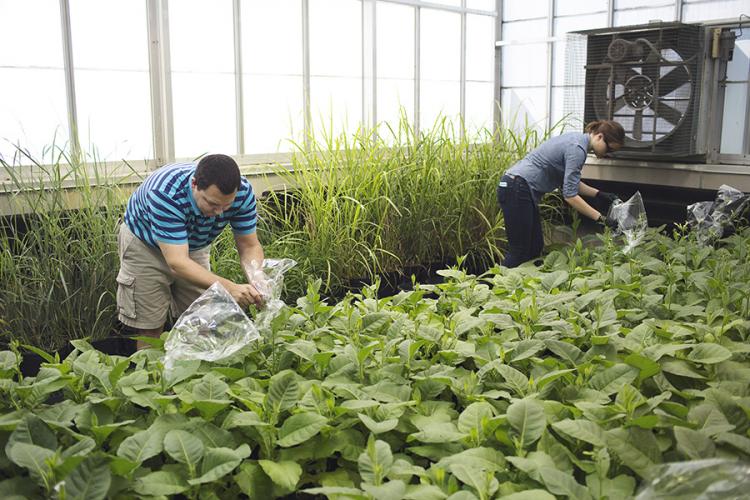
University of Illinois researchers Paul South (left) and Caroline Keller (right) bag tobacco plants that have set seed in the greenhouse. They are in the process of sifting through 1500 plants to identify those with the genetically engineered changes that the scientists seek.
CREDIT: HALEY AHLERS/UNIVERSITY OF ILLINOIS
The limits of good breeding
Traditional crop-improvement tools have already led to extraordinary leaps in yield. Starting as far back as the 1920s, plant breeders created plants that not only grew faster, allowing for more plantings per season, but also produced more calories for eating. Those gains, part of a larger agricultural initiative known as the Green Revolution, were partly achieved by selecting for two traits: plants with leaves that would intercept as much light as possible and plants that would funnel as much biomass as possible into the edible seeds. Today’s premier varieties of soybeans capture nearly 90 percent of available sunshine and pack as much as 60 percent of their biomass into the bean; wheat and rice also saw hefty boosts in yield.
But the gains are approaching a ceiling — a plant can’t be nothing but seed, after all. Where might crop scientists turn? The inefficiencies of photosynthesis are an obvious choice, says Don Ort, a plant biologist at the Urbana-Champaign campus. The Green Revolution didn’t tackle this feature. Conventional plant breeding harnesses natural variation, seeking out individual plants with traits of interest: slightly larger seeds than their neighbors, for example, or significantly shorter stems. But that strategy doesn’t work for photosynthesis, Ort says. Most plants’ photosynthetic machinery chugs along at pretty much the same rate — there isn’t a spectrum of lousy to superstar to select from.
Still, research that would lay the groundwork for confronting those inefficiencies was underway at the University of Illinois many decades back. In 1965, the United States Department of Agriculture hired William Ogren to work at the campus and look into improving soybean photosynthesis. The mild-mannered chemist would end up doing groundbreaking research that set off some fiery years in plant biology and saw more than one academic meeting devolve into shouting matches.
Ogren — and much of the plant physiology community — was intrigued by what appeared to be a major glitch in photosynthesis. Under certain circumstances, plants produced a toxic compound called glycolate. Plants make it, but because it’s toxic they then have to dismantle it. Making and then cleaning up glycolate — a process called photorespiration — is a major waste; it leads to chemical reactions that release valuable carbon back into the air. So what caused the plants to make the glycolate in the first place? Ogren and his post-doctoral researcher George Bowes discovered the culprit: the enzyme in charge of one of the first steps of photosynthesis. Known today as rubisco (shorthand for ribulose-1,5-bisphosphate carboxylase/oxygenase), this enzyme’s job is to grab carbon dioxide that enters the leaf and tack it onto another molecule in the assembly line. It turned out that the inept enzyme will happily grab oxygen instead, sending it along in carbon dioxide’s place. And when rubisco passes oxygen into the photosynthesis assembly line, glycolate results, gumming up the works.
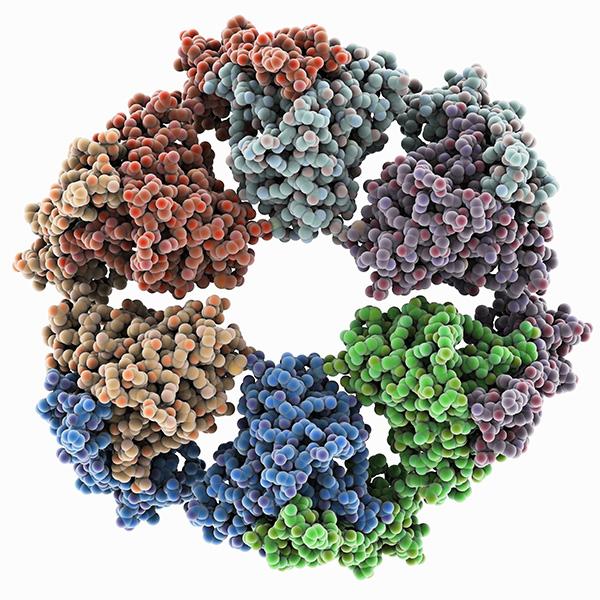
Molecular structure of rubisco, a pivotal enzyme in photosynthesis. It captures carbon dioxide that enters the leaf — but sometimes captures oxygen by mistake. Rubisco’s sloppiness wastes a lot of energy.
CREDIT: LAGUNA DESIGN/SCIENCE SOURCE
Rubisco’s mistake is costly. Estimates suggest that photorespiration can reduce the efficiency of photosynthesis by more than 40 percent. “If you designed that, it would be considered an engineering failure,” says biochemist Sabeeha Merchant of the University of California, Los Angeles.
Yet rubisco’s sloppiness today is rooted in its ancient origins and stunning past success. It is ubiquitous — possibly the most abundant protein on Earth. Plants, algae and light-harvesting bacteria all depend on it for turning inorganic carbon into usable organic matter. It is responsible for more than 99 percent of global primary production, that remarkable process whereby biomass emerges from thin air.
Billions of years ago, when rubisco began doing its job, there was barely any oxygen in the air. It didn’t matter if the enzyme picked up the occasional oxygen molecule — there wasn’t much of the stuff around. Rubisco’s life work, photosynthesis, changed that. Over the eons, it pumped more and more oxygen into the atmosphere. Today, atmospheric oxygen is roughly 500 times more abundant than CO2. That oxygen is rubisco’s Achilles’s heel: Though precise numbers vary depending on environment, broad-brush calculations suggest that for every five carbon dioxides, rubisco grabs two oxygen molecules. And rubisco could not evolve later on to fix its biochemical promiscuity: Scientists surmise that its evolutionary journey had boxed it in, such that minor mutations would knock out its function altogether.
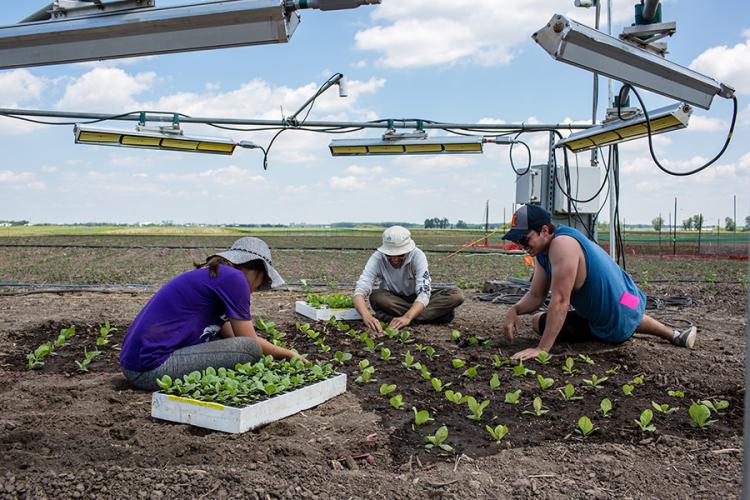
Scientists plant tobacco in SoyFACE (Soybean Free Air Concentration Enrichment), an outdoor laboratory that allows them to manipulate temperature and carbon dioxide levels and see how the plants fare under different environmental conditions.
CREDIT: CLAIRE BENJAMIN/UNIVERSITY OF ILLINOIS
“When we think about plants, we forget that they’ve been around for so long,” says Amanda Cavanagh, a University of Illinois post-doc, as we descend in an elevator toward an underground tunnel that serves double duty as a campus tornado shelter and a quick shortcut between buildings. “Their machinery can’t cope with the current high-oxygen environment, the environment they shaped. It’s a problem for the plant, and it’s a problem for the food system.”
A genetic bypass
I’m in the tunnel with Cavanagh and Paul South, a molecular biologist with the US Department of Agriculture, headed to the tobacco seedling torture chamber. The tunnel is well-lit and clean, though South gleefully points out a budding stalagmite on the floor, the result of a slow drip through a ceiling crack. Somewhere above us, a few hundred yards of dirt away, is the greenhouse where Ogren grew his soybeans decades earlier.
Six-day-old seedlings sprout perkily in a tray next to the torture chamber, unaware of their future. The box is a crucial first test after months of genetic tinkering by South. If his efforts are successful, some of those baby tobacco plants won’t mind the dangerously low levels of carbon dioxide they’re being subjected to. They contain a genetic workaround, a bypass that will compensate for rubisco’s cursed affinity for oxygen. Their photosynthesis machinery will keep humming even as neighbor seedlings without the bypass ultimately wilt and die, their chlorophyll ravaged by the toxic product of rubisco’s mistake.
Photorespiration is a convoluted process, akin to trekking through three different buildings to get waste to the curb. The glycolate is modified piecemeal. It is made in the chloroplast, then transits through two other cellular compartments, the peroxisome and the mitochondrion. Some of its carbon is recycled along the way and sent back to the chloroplast to make sugars, but the effort still adds up to loss. The idea behind the scientists’ bypass is to modify the plant’s genetic material and so recycle glycolate immediately — right in the chloroplast — recovering all the carbon and saving energy. “Photorespiration takes the country roads,” says South. “The bypass is like installing a freeway.”
The idea has a proven track record. A decade ago, for example, researchers led by plant biologist Christoph Peterhänsel, then at RWTH Aachen University in Germany, successfully created a photorespiration bypass in the weedy mustard Arabadopsis, the plant-biology equivalent of the lab rat. Their approach, reported in Nature Biotechnology in 2007, borrowed genes from the bacterium Escherichia coli to streamline the glycolate cleanup. The plants responded, growing faster and producing more shoots and roots than their ordinary counterparts.
Another approach, led by plant biochemist Veronica Maurino of Heinrich Heine University in Germany, used genes from both plants and E. coli. Published in 2012 in Frontiers in Plant Science, that work also led to enthusiastic growth. But these past efforts didn’t use the tools available to today’s genetic engineers, which make it possible to insert several desirable genes in a row, and to include bits of DNA that can dial up or down the activity of the inserted genes.
Also in the years since, researchers have discovered two more proteins to play with. Their job is to ferry glycolate out of the chloroplast, allowing the by-product to escape. The Illinois scientists have tools to inactivate these, in what’s now a full-court anti-photorespiration press.
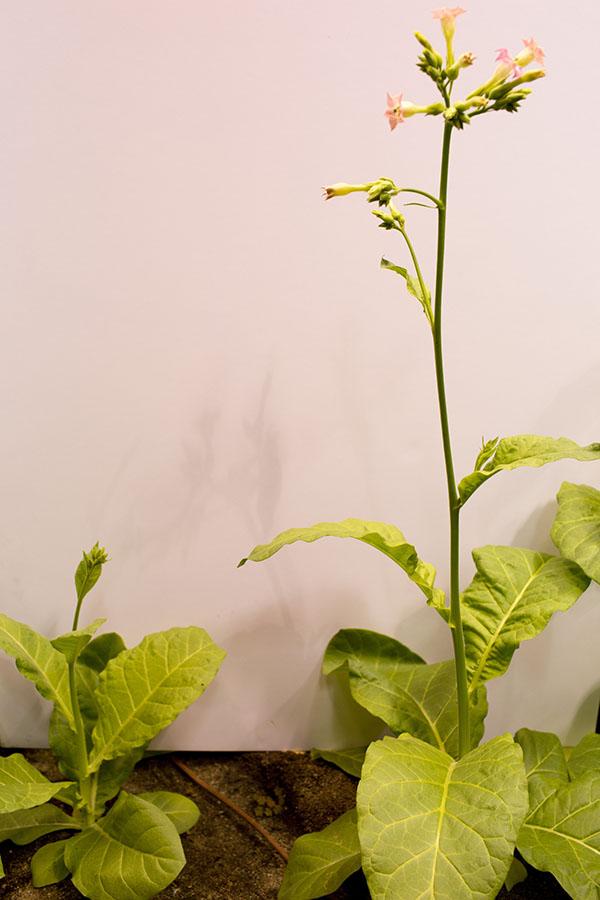
An unmodified tobacco plant (left) is dwarfed by one the same age that has been genetically modified to bypass the inefficiencies of photorespiration (right).
CREDIT: CLAIRE BENJAMIN/UNIVERSITY OF ILLINOIS
The Illinois work also adds a grand computational twist. Rather than deciding which precise genes to modify and how, South has used computer programming to generate 24 potential designs that mix and match the bypass machinery. They cover an array of alterations: new arrangements of already-tested bypass genes; genes snipped from different sources such as algae; and sundry DNA switches to turn various genes on and off (or dial photorespiration back up if it turns out to be more important than scientists assume).
The end result of all this shuffling is 140 genetically distinct tobacco plant lines with distinct bypass designs. Each will undergo a battery of tests, including greenhouse and field trials. Maurino, whose early bypass research helped fuel the current work, is confident in the approach. “Their results seem very promising,” she says. “I’m very excited to hear more.”
$[$PB_DROPZONE,id:donate-promo$]$The researchers may not end up with one “best” solution — in fact, they don’t want to, because real-world environments vary greatly. Working with several bypass designs will allow them to identify versions that would excel in, say, drought conditions, nitrogen-poor soils or very hot temperatures. That would be the mark of success: ramped up photosynthesis in a true crop plant, grown the way a farmer might grow it. The current work modifies a cigar variety of tobacco called Petit Havana, but soybean, potato, and cowpea — a staple in sub-Saharan Africa — are all on the horizon.
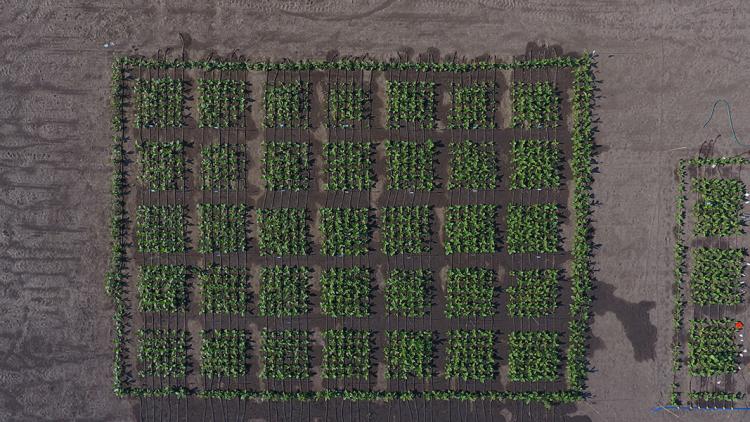
Aerial drone shot of tobacco during a 2017 experiment to test plants that were engineered to bypass photorespiration.
CREDIT: BEAU BARBER/UNIVERSITY OF ILLINOIS
Green machines?
Early results are encouraging. I’m taken to one of the lab greenhouses and the bypass plants are easy to pick out, with flowering shoots that stretch above the fledgling greenery of their ordinary neighbors. In field trials conducted in 2016, tobacco plants with a bypass had 18 to 20 percent more biomass — the added heft in leaves and stems — than ones that hadn’t been tinkered with (the results will be published early in 2018). They also flowered earlier, which could enable two plantings a year, Cavanagh explains. She is testing the plants to see how different versions of the bypass handle different environmental conditions, taking advantage of a unique university field station where you can manipulate temperature and gas concentrations in plants grown outdoors. She notes that people often assume that the higher carbon dioxide levels of the future will be better for plants, but this isn’t necessarily the case (see sidebar).
Researchers aren’t putting all their seeds in one basket, though. The bypass project is just one prong in a larger international initiative called RIPE (Realizing Improved Photosynthetic Efficiency), headquartered at the Illinois campus and with collaborators at the University of California, Berkeley; Louisiana State University; and institutions in Australia, the United Kingdom and China. RIPE is targeting weak links and bottlenecks all along the roughly 160 steps of photosynthesis, supported by $25 million from the Bill and Melinda Gates Foundation and $20 million more from the Foundation for Food and Agricultural Research and the UK's Department for International Development. The ultimate goal is to increase the output of staple food crops.
There’s an urgent need to push this work along, says University of Illinois crop physiologist Steve Long, director of RIPE. Revving up the various sluggish spots in the photosynthesis pathway and bringing them all together through a mix of genetic engineering, computer modeling and conventional plant breeding will be a long, slow process. And though it might be hard for Americans to conceive of a world short of calories, the forecasted population increases, combined with global warming and their heavy toll on the environment may mean more expensive food in many parts of the world. “Whatever we invent today is not going to be available for 20 years or more, so we need to be looking now at what are the best technologies we can put on the shelf,” Long says.
Dealing with rubisco’s shortcomings is one major focus. In addition to the bypass project, researchers at RIPE and elsewhere are working on ways to swathe rubisco with CO2 so it doesn’t encounter oxygen to begin with, and scouring algae and wild plants for versions of rubisco that stay on task, ignoring oxygen.
The team is also tackling aspects of photosynthesis that aren’t the fault of rubisco but of inefficient use of light. When sunlight gets too strong, plants shed the extra energy as heat, to avoid damage. This light-quenching mode can take minutes to hours to turn off, even after clouds have moved in — a major waste. To fix the problem, Long and his RIPE colleagues added genes to tobacco plants to speed the bounce-back. The strategy, reported in Science in 2016, upped the amount of biomass in plants by 14 to 20 percent.
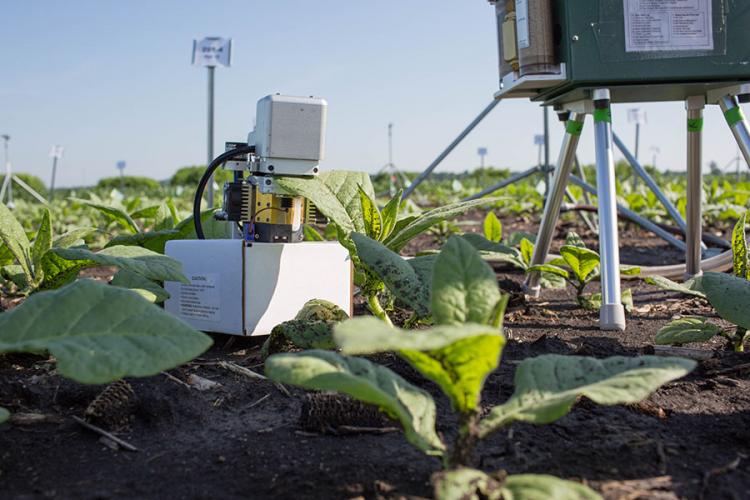
In a 2016 field experiment, an infrared gas analyzer measures carbon dioxide intake — and thus photosynthesis rate — of plants genetically engineered to bypass photorespiration.
CREDIT: CLAIRE BENJAMIN/UNIVERSITY OF ILLINOIS
Other scientists outside of RIPE are tackling the photosynthesis problem by trying to mimic a strategy that has evolved numerous times in the natural world. Roughly three percent of land plants use a different enzyme, one that ignores oxygen, to snatch up CO2. These “C4” plants, which include crabgrass, sugarcane and corn, have a very different anatomical structure that keeps rubisco away from oxygen. (Rubisco still has jobs later on in the photosynthesis assembly line.) Such plants have very low levels of photorespiration. Inspired by this, a team led by plant developmental geneticist Jane Langdale at the University of Oxford in England are trying to engineer a C4 version of rice. The project, funded by the Gates foundation, is complex, but in a solid step toward that goal, the scientists reported this November in Current Biology that they’d engineered anatomical tweaks to bring rice closer to C4 anatomy.
Of course, science won’t be enough to feed the world of the future, says Fischer of the Commonwealth research organization. It’s also going to take policy changes and basic infrastructure improvements, such as roads and electricity, in developing parts of the world. “All of these have to be fixed as farmers embrace new technologies, and that means huge changes in public and private investment and in governance,” Fischer says.
Those are gargantuan challenges. But in the hopes they will be met, researchers are doggedly torturing one generation of plants at a time and seeing what bears fruit.