In May of 2011, a savage epidemic of fooARrne illness broke out in Germany. Health officials quickly identified the culprit as a gut-poisoning strain of the bacterium Escherichia coli, but it took more than a month to trace its source to contaminated bean sprouts grown from fenugreek seeds. By the time the epidemic burned itself out many weeks later, cases had spread to several countries in Europe and North America, close to 4,000 people had been sickened, and 53 had died. Some 850 developed a life-threatening complication called hemolytic uremic syndrome, or HUS, in which Shiga toxin produced by E. coli ravages the kidneys, causing acute kidney failure.
Adrienne and James Paton, husband-and-wife researchers at the University of Adelaide in Australia, watched the outbreak unfold from the other side of the globe, convinced they could have limited the extent of the fallout. A little more than a decade earlier, Adrienne Paton had dreamed up a way to treat the disease by mopping up all the Shiga toxin through a clever trick. The damaging toxin, she knew, binds to certain sugar molecules on the surface of human cells. What if one were to engineer a harmless species of E. coli that naturally resides in the gut to make those sugars too, and display them on their surface? The altered microbes, now studded with these Shiga receptors, might sop up the toxin, thereby sparing the human body from assault.
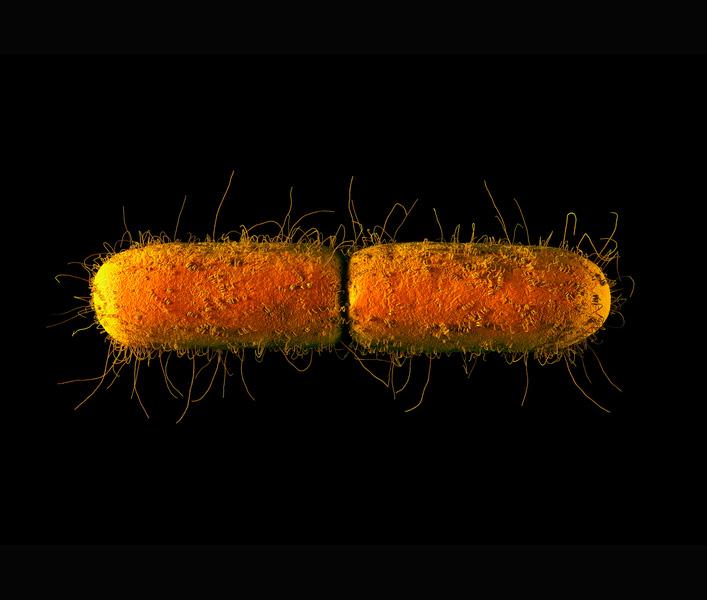
The bacterium Escherichia coli (computer illustration shown) usually lives a quiet life in the gut of humans and other animals. Some strains go rogue and cause disease, but others have proved helpful. Now, scientists are using bioengineering to harness the power of beneficial strains like E. coli Nissle 1917 and other probiotics to battle a range of different diseases.
CREDIT: GETTY SCIENCE PHOTO LIBRARY
The strategy seemed to work: Thirty-two mice infected with the two most toxic forms of Shiga-producing E. coli that the microbiologists could find recovered completely when given this engineered probiotic, the Patons reported in a 2000 study. “Instead of 100 percent death, you had 100 percent survival,” James Paton says. The researchers engineered a similar toxin-mopping approach to treat cholera, and it, too, worked well in animal models.
The duo patented the approach, but couldn’t find investors willing to fund efforts to take it to human trials, Paton says. For one thing, there was little appetite back then for the idea of ingesting genetically engineered bugs, in part because of safety concerns and in part because regulators had no framework for evaluating them. But even more problematic, he surmises, was the fact that while HUS pops up prominently during E. coli outbreaks, the total number of people affected is small and the market for such a medicine would be limited. “We were disappointed that we didn’t get traction — very much so,” Paton says.
The Patons’ idea withered — but today, the concept of engineering probiotic microbes to make medicines seems poised to hit the big time. Molecular biology techniques for genome engineering have been around since the 1970s, but the process back then was cumbersome. In the last 15 years, powerful tools have emerged for designing gene circuits to perform specific cellular functions, such as churning out proteins that can act as medicines. Researchers have also come up with techniques that prevent these living drugs from functioning outside the human body, easing issues around safety.
Several academic research groups and about half a dozen companies are now working on engineering gut bacteria along these lines. Some of the tinkered-with bugs serve as detectors, producing a fluorescent protein when they encounter molecules indicating inflammation or cancer in the body. Others are being put to work pumping out proteins that scavenge or deactivate toxins, like the Patons’ E. coli did, or that perform a healing function themselves. “With engineered bacteria that operate from the gut, you can perform a lot of therapeutic actions,” says Jose-Carlos Gutiérrez-Ramos, president and chief executive officer of the Cambridge, Massachusetts-based company Synlogic.
It started with yogurt
The idea that ingesting beneficial gut microbes can promote health goes back more than a century, when the Russian zoologist Elie Metchnikoff observed that peasants who drank fermented yogurt enjoyed longer, healthier lives. Metchnikoff’s work inspired the Japanese microbiologist Minoru Shirota, who isolated a strain of gut bacteria, Lactobacillus casei, that could stave off pathogens. In 1935 Shirota founded a company called Yakult, which still makes a dairy beverage that contains it. It wasn’t until the 1960s, though, that the term probiotics emerged to describe beneficial gut bacteria, and it took another couple of decades for the concept of using probiotics therapeutically to take hold.
Today, researchers are beginning to gain a broader understanding of the community of microbes that dwell inside us (what is called the gut microbiome) and the significant role it plays in an enormous range of disorders, from inflammatory gut maladies such as Crohn’s disease and metabolic conditions such as obesity and diabetes, to neurological and neuropsychiatric disorders including multiple sclerosis, autism and depression.
But despite the commercial success of a $35-billion-and-growing probiotics industry that sells a dizzying array of food supplements, and microbially spiked foods and beverages for humans and animals, so far few studies have clearly shown that modulating the gut microbiome with probiotics is effective. Some researchers argue that engineering microbes to perform more specific, targeted functions may enable them to pack a bigger punch.
“You can target specific molecules that you want to be degraded or produced in the gut and add those activities into the bacteria that are naturally abundant in the gut,” says Weston Whitaker, cofounder of Novome Biotechnologies, a start-up based in South San Francisco, California, developing therapeutics with this approach. “Because we are engineering these activities, we think we can do it in a much more predictable and extensible way than if we were using natural species.”
Indeed, propelling this therapeutic strategy forward are unprecedented advances in biological engineering and synthetic biology, a field dedicated to creating a tool kit of standardized genetic components for use in redesigning living creatures. Scientists know much more than they did a few years ago about mixing and matching genes — often synthesized from scratch — and delivering them to cells. These form genetic circuits that produce new molecules, hobble the production of ones normally there, detect chemical signals or otherwise regulate the cell’s activity. “The synthetic biology revolution over the last five years has been embraced by people in the probiotic environment, and that’s led to a huge amount of interest,” says Roy Sleator, a molecular biologist at the Cork Institute of Technology in Ireland.
Timothy Lu, a synthetic biologist at the Massachusetts Institute of Technology, recalls that when he was doing his PhD 10 years ago, it would take him about three months to engineer a single such circuit. “Now my graduate student can do 20 of these in a week, and that allows people to take a potentially interesting circuit and optimize it like crazy,” he says.
Progress on probiotics
It’s still early days — no genetically engineered microbial therapies have yet hit a regulatory home run. Perhaps the earliest partial success story came from work that engineered the bacterium Lactococcus lactis, which is widely sold and consumed as a probiotic. By inserting the gene for this protein into the microbial genome, scientists prodded L. lactis to produce the anti-inflammatory molecule interleukin-10. In research published in 2000 — the same year as the Patons’ study — molecular microbiologist Lothar Steidler and his team at the University of Ghent in Belgium showed that the altered microbes, when eaten, can halve gut inflammation in mouse models of inflammatory bowel disease.
Three years later, Steidler’s team added a twist — they redesigned their circuit to work only in the presence the molecules thymine or thymidine, which are abundant inside the human body but scarce outside of it. This blocked the microbe’s ability to spread its engineered genes if released. With that safety feature, Steidler and his colleagues established a company called ActoGeniX (which was recently acquired by Intrexon Actobiotics) to test the medicine in human trials. But although the treatment was shown to be safe, it did not benefit patients with inflammatory bowel disease. It’s unclear why, says Lu — the microbes might not have been churning out enough IL-10 to quiet the disease in the human gut, or perhaps it was not the right treatment. “That example did prove safety, though, and how to get into clinical trials,” he says.
Several researchers since then have worked with other probiotic microbes, such as Lactobacillus or E. coli. Strains of both reside harmlessly in the gut, and are also sold commercially as probiotics, so they are well-tested and known to be safe.
The added benefit for E. coli is that microbiologists and synthetic biologists have studied it intensively for decades and have developed a wide array of tools for genetically modifying it. But whatever the microbe, the circuits that researchers are adding generally come from recombining genes that the organism already has.
Infectious diseases are an especially promising area for therapies using engineered probiotics. That’s because pathogens commonly pump out some sort of signaling molecule that next-gen probiotics can be programmed to target, says Matthew Chang, a biochemist and synthetic biologist at the National University of Singapore. This year, Chang and his colleagues engineered the E. coli strain Nissle 1917, which is marketed to consumers as a probiotic, to produce one protein that detects the presence of the pathogenic bacterium Pseudomonas aeruginosa, and two enzymes that — in a one-two punch — break apart the biofilm that the organism builds to protect itself and kill individual cells.
Mice infected with Pseudomonas aeruginosa and given the probiotic were effectively treated. When they ate the probiotic before being infected, the pathogen didn’t sicken them. “We can envision these reprogrammed microbes as a preventative measure, let’s say for people at high risk of contracting an infectious pathogen,” Chang says.
Other health applications can also be targeted. Cornell University biological engineer John March, who was one of Chang’s colleagues on the P. aeruginosa study, in 2015 created a strain of Lactobacillus that secretes a substance called GLP-1(1-37), which coaxes cells lining the intestine to produce insulin. Diabetic rats that ate the altered microbe were able to replace 25 to 33 percent of their insulin.
Probiotics could also tackle disease diagnosis. Researchers in 2015 reported creating E. coli Nissle strains that could detect tumors or high glucose levels in urine by releasing a luminescent or a red fluorescent compound, respectively. And synthetic biologist Pamela Silver’s lab at Harvard University has added genes into E. coli that enable it to detect inflammation by sniffing out a substance — tetrathionate — that’s produced by inflammatory cells, and to survive for some six months in the mouse gut. “Our sensor system is quite robust, and we show that it can be adapted to many things, like markers of stress, nutrition or colon cancer,” she says.
Doing what the body can’t
These efforts chug along, mostly under investigation in cultured cells or mice. But one E. coli Nissle 1917-based therapy is already in clinical trials. The live-bug drug, called SynB1020, is under development by Synlogic, which was founded in 2014 by Lu and synthetic biologist James Collins, another MIT researcher. The scientists designed it to treat a rare constellation of genetic and chronic conditions called urea cycle disorders in which nitrogen — a waste product generated as proteins are broken down — builds up in the blood in the form of ammonia. Normally, the body converts ammonia to urea and flushes it out of the body in urine, but a malfunction in this cycle causes urea buildup, brain damage and death. SynB1020 is E. coli Nissle engineered with a series of genes that scavenge ammonia and transform it into the harmless amino acid arginine.
Scientists put SynB1020 through its paces in early-stage tests that plied 52 healthy volunteers with the probiotic in the form of a liquid. In November, based on the findings, the company reported that the product was safe. Early in 2018, it plans to begin safety and dosage-level tests in patients with cirrhosis of the liver who have chronic elevated ammonia. In mid-2018, trials will expand include patients with genetic urea cycle disorders.
The company also plans to begin tests on a second therapy targeting a rare genetic condition called phenylketonuria, or PKU, which causes mental retardation if patients aren’t treated from early life with an extremely low-protein diet. In this second therapy, SYNB1618, the engineered E. coli Nissle carries a protein on its surface that sucks up the amino acid phenylalanine, which PKU patients can’t process.
“The concept is, if you with your liver or kidney or pancreas cannot do a certain metabolic function like degrading a toxin or producing a metabolite, we engineer this probiotic bacteria to do it,” says Synlogic chief executive Gutiérrez-Ramos. “We add that function to the microbiome.”
Researchers are still figuring out which types of microbes might work best for such therapies. Probiotics like E. coli Nissle are commercially available and safe, but they generally pass through the gut without colonizing it, Lu says. He and other researchers are also engineering commensal gut bacteria, which are present in the gut long-term. Therapies developed from these, as opposed to more transient species sold as probiotics, like E. coli Nissle and Lactobacillus lactis, may have more staying power and thus stronger effects, he explains.
His lab has focused on a genus of bacterial species called bacteroides, which together make up about 25 to 30 percent of the microbial population in the gut. In 2015, they altered a particular species, Bacteroides thetaiotaomicron, making it more engineerable by inserting a set of genetic elements that can switch genes on and off under specific circumstances — readying it to do whatever therapeutic tasks are engineered into it. Bacteroides is also the genus of choice for Novome Biotechnologies’ Whitaker.
A complicated endeavor
Creating “designer” microbe therapeutics sounds simple: In theory, gene circuits can be slipped into any old microbial genome like Lego bricks. And to some extent they can — modularity is certainly the goal. Nonetheless, researchers face a long list of challenges.
For one thing, microbial genomes have evolved to contain just the functions they need to thrive in an environmental niche, and adding novel genes can destabilize this balance. That’s especially so if a circuit is complicated and leads to any growth defects, Silver says. Later generations of the bug may simply evolve away from the human-made alteration. That might not matter for a very short-acting therapy, but it won’t fly for anything meant to linger for a significant length of time. There are ways to stably maintain added functions, though they involve some genetic trickery.
Getting an added gene to be active enough for therapeutic effect also requires significant fiddling, says Lu. And if the gene circuit you’re adding consists of more than one function, they may interact in unpredictable ways. “It isn’t like 1 plus 1 plus 1,” Lu says. “Sometimes things break and you don’t know why.”
Then there’s the fact that the gut is a chaotic, fast-paced and quickly changing environment. Once within it, the carefully engineered microbe will find itself surrounded by thousands of other microbial species, all going about their metabolic business, as well as human cells performing their own set of functions. Interactions in that stew can be precarious. What’s more, anything ingested moves through the gut at a clip of about one centimeter per hour; many different chemicals are distributed in this tunnel-like environment, and pH fluctuates throughout. Microbes must be robust to survive the trip.
In fact, because of such unknowns, some scientists don’t think we’re ready yet for next-generation probiotics. “Engineering is a science that kicks off when we have a good knowledge base,” says Martin Fussenegger, a synthetic biologist at ETH Zurich in Switzerland. He notes that researchers still know very little about the composition of the microbiome, much less the function and interactions of its myriad denizens and how they affect their human hosts. “There is so much information still to be found, interpreted and implemented before starting to engineer gut microbes.”
And that includes some of the issues that gummed up the prospects for the Patons’ cholera and food-poisoning work in the early 2000s: concerns about safety. “Once our microbes go into the body, there is no way we can really contain them,” Chang says. That makes questions of biocontainment and safety paramount. In the past few years, researchers have developed a handful of strategies for making sure that bioengineered bugs don’t escape the body and colonize the environment. They routinely knock out genes essential for growth; they might also program a feature into a circuit, like Steidler’s team did, that makes bacterial survival dependent on a molecule present exclusively in the human body, or stops bacteria from dividing when exposed to molecules outside of it — that is, a kill switch.
There are strong signs that outright rejection of genetically modified medicines is on the wane — both by regulatory agencies such as the US Food and Drug Administration, and by the public, at least in the United States. One hint of this change is the recent approval of two cancer therapies in which patients’ immune cells are modified. And researchers argue that for many engineered probiotics, containment is not a huge concern. “We don’t use crazy bacteria — we use a probiotic that you can buy without a prescription in Europe, Canada and Australia,” says Gutiérrez-Ramos. “We truly believe that what we call ‘synthetic biotic medicines’ will be among the armamentarium of therapies that our society will have in the future.”