Living things just seem to know how big to grow — and how big to grow their sundry parts. A human liver maintains itself at just the right volume to do its job. A fruit fly’s wings, on opposite sides of its body, somehow wind up the same size as each other, correctly scaled to sustain flight.
In everyday life, we expect body parts to be in proportion, because they usually are. “You notice if somebody comes up in front of you and one leg is way bigger than the other,” says Ben Stanger, a gastroenterologist and researcher at the University of Pennsylvania’s Perelman School of Medicine who has studied organ growth.
But as much as we take this basic aspect of life on Earth for granted, scientists don’t fully understand it. How do body parts know when to start and stop growing?
In some cases, cells seem to follow an intrinsic program carried out by the activity of their genes. At other times, cells appear to react to a cacophony of messages they receive from other cells and their environment, turning growth on and off as needed.
A lot of times, they seem to do a little of both. And when they’re cancer cells, the whole business has gone awry.
“We don’t get it,” says Stanger, author of a 2015 article in the Annual Review of Physiology that described mechanisms that control liver growth.
The sizes that organs and other body parts reach can be set internally, inside the tissues of those body parts (blue), or controlled by the environment (green). Here are some examples.
Starting with salamanders
Scientists have been trying to “get it” for a long time. In the 1930s, Yale zoologists Victor Twitty and Joseph Schwind conducted experiments in salamanders, cross-transplanting limb buds from a smaller species, Ambystoma punctatum, with those of a larger but closely related species, Ambystoma tigrinium. In some experiments, the researchers found that taking a limb bud from the small salamander and grafting it onto the larger salamander resulted in an animal with three large limbs and one small one (and vice versa). This suggests that “the information for size was embedded in that group of cells very early on and didn’t care what was happening in the animal,” Stanger says.
But Twitty and Schwind found in other experiments that nutrition — an external regulator — also affected limb size. “It’s nature and it’s nurture,” Stanger says. “In biology, it’s never either/or.”
Developmental biologists soon discovered a variety of ways that organs and structures achieve their ultimate sizes. In one famous 1960s experiment, researcher Donald Metcalf implanted 6 or 12 fetal mouse spleens into individual adult mice whose own spleens had been removed. He found that each implanted spleen grew to a proportional fraction of the size of a normal adult spleen — leaving the animal with a normal total amount of spleen material. This suggests that spleen tissue has a way of understanding how much of it there is in relation to the body, says Jamie Davies, an experimental anatomist at the University of Edinburgh in Scotland. But — “really annoyingly,” Davies says — Metcalf also found that multiple thymus grafts implanted in an adult mouse behave completely differently: Each grows to its full adult size.
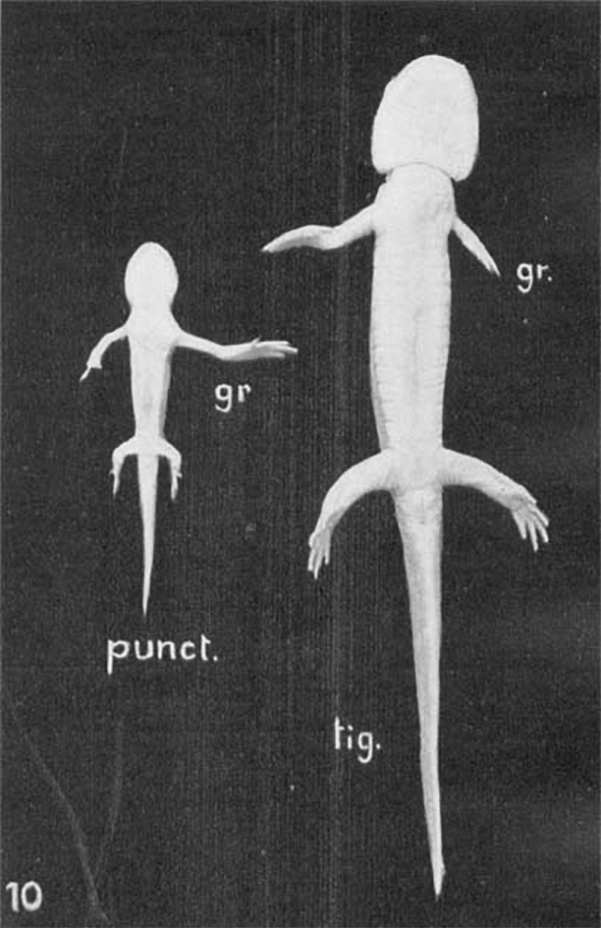
In a classic 1930s experiment, scientists took limb buds from a small salamander species and transplanted them onto a larger salamander species, and vice versa. In results shown here, the size of regenerated limbs was controlled by the transplanted tissue, not the recipient. Somehow, salamander limb buds seem to “know” what size they should grow to.
CREDIT: V.C. TWITTY & J.L. SCHWIND / THE JOURNAL OF EXPERIMENTAL ZOÖLOGY 1931
Decades later, Stanger found similar growth differences in the mouse liver and pancreas: Cells that give rise to the liver use environmental cues to determine how much the developing organ should grow, while those that form the pancreas follow an “autonomous trajectory” — they always achieve a preprogrammed size, no matter what is going on around them.
Pumping the brakes on growth
Scientists have sussed out a reasonable amount of detail about some of the feedback-based programs that direct growth. A protein called myostatin, for instance, helps to suppress muscle growth. When the tissues get large enough to pump out a threshold amount, muscle cells stop growing. The molecular processes that dynamically regulate liver size seem to involve tissues in the gut: When levels of bile acid fall (a sign of reduced liver function), those gut tissues produce factors that disengage a brake on liver growth known as the Hippo pathway. As a result, growth kicks into gear — allowing the liver to grow to its proper size. When bile acid levels rise to normal, Hippo comes back on, and liver growth turns off again. And so on.
The Hippo pathway is a super-popular subject of study today, both because of its job in regulating organ size and because of its potential role in controlling cancers. Many questions about it remain unanswered.
Mysteries also remain for cases where instructions for size are baked in, as seen in those early experiments with salamander limbs, says Laura Johnston, a geneticist and developmental biologist at Columbia University Medical Center. Labs are delving into a number of inputs that may play a role in directing cells to grow, from information about cell fates and cell organization that are hardwired in the DNA, to mechanical forces on tissues.
An experiment in the 1960s showed that implanted mouse spleens can sense how much other spleen tissue is around and limit the size that they grow to. The result is a mouse with the right amount of spleen material. On the left is a mouse with a normal-sized spleen. On the right is a mouse whose own spleen was removed before transplantation with six pieces of spleen tissue.
Johnston’s own research, some of which she and her coauthors described in a 2009 article in the Annual Review of Cell and Developmental Biology, focuses on a phenomenon known as cell competition — interactions that lead to the deaths of unfit or unneeded cells. It seems to play a role in stabilizing organ size. When researchers in her lab blocked cell-death mechanisms in the cells that give rise to fruit fly wings, they found that the bell-curve-shaped distribution of wing sizes normally seen in fly populations broke down. A larger-than-usual number of flies developed overly large wings, or overly small ones. It’s as if, she says, “the precision of size regulation is lost if the cells can’t do these competing interactions.”
There’s still much to learn out about the deceptively simple, fundamental questions of how an arm matches its corresponding limb or how a liver ends up just the needed size. But the questions have practical ramifications too. Many growth studies today, including myriad explorations of the Hippo pathway, are conducted in the service of understanding and treating cancers. “Researchers are saying, ‘Look — cancer is development gone wrong, and it’s obviously growth-connected, so we really need to understand growth on its own,’” Davies says.
Also interested in organ growth are researchers who want to engineer tissues using stem cells. “There’s always the worry that if you build something that will grow up inside the body,” Davies says, “will it know when to stop?”