In 1916, Antarctic explorer Ernest Shackleton was forced to make a treacherous voyage across the open waters of the Southern Ocean after his ship Endurance was crushed by pack ice and sank in the Weddell Sea. Along with five members of his party, Shackleton set out for the island of South Georgia in a 23-foot-long wooden lifeboat named the James Caird. Eleven days into the trip, on May 5, Shackleton thought he spied a clearing in the murky sky above. What he was actually seeing wasn’t a break in the clouds, but the white crest of a monstrous wave bearing down on them.
“During twenty-six years’ experience of the ocean in all its moods I had not encountered a wave so gigantic,” Shackleton would later write in his book South: The Story of Shackleton’s Last Expedition 1914-1917. “It was a mighty upheaval of the ocean, a thing quite apart from the big white-capped seas that had been our tireless enemies for many days.”
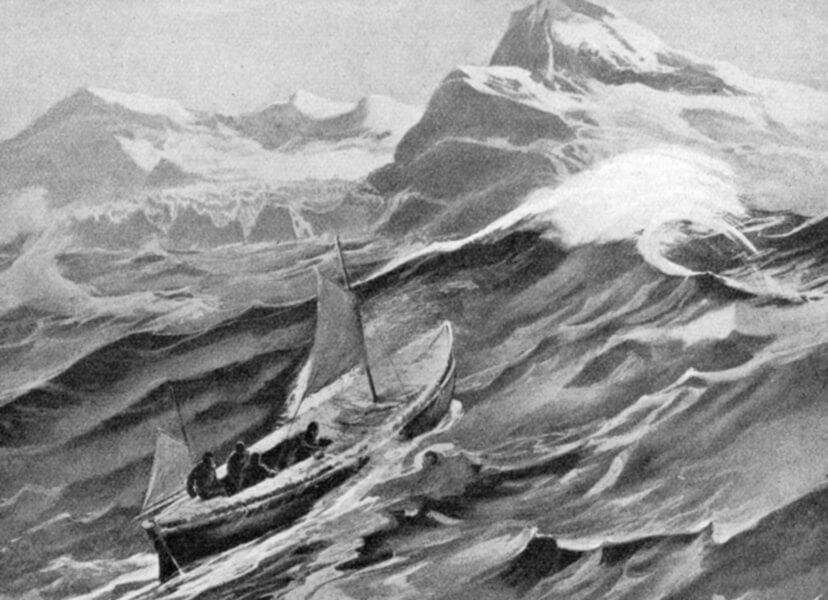
This illustration depicts the horrendous waves and weather that Ernest Shackleton and five shipmates endured as they journeyed 800 miles across the Southern Ocean, from Elephant Island to South Georgia, in the dinghy James Caird. It was published in the 1925 book Argonauts of the South, by expedition photographer Frank Hurley.
CREDIT: INTERFOTO / ALAMY STOCK PHOTO
Shackleton’s accountremains one of the most evocative descriptions of the westerly winds that circle the globe between 30 and 60 degrees latitude in both hemispheres and the colossal waves they whip up in the Southern Ocean. Today, the southern westerlies are back in focus for entirely different reasons: their potential impact on climate change.
The world’s oceans take up more than a quarter of the carbon humans are emitting into the atmosphere, partially mitigating the greenhouse effect of that carbon. And the Southern Ocean accounts for nearly 40 percent of this marine carbon absorption, even though it makes up only one-fifth of Earth’s ocean surface area. There could be enormous consequences for our already warming planet from even a small reduction in the Southern Ocean’s ability to suck up carbon from the atmosphere.
Scientists say this reduction may already be happening, and they suspect that the westerlies in the Southern Hemisphere, which are stronger than their northern counterparts because they blow mostly over open water, are to blame. Records of actual wind speeds, as well as estimates of wind speeds from measurements of atmospheric pressure, provide clear signs that the westerlies in both hemispheres are shifting toward the poles and intensifying. Climate models show that these changes are partly due to global warming, and research is afoot to determine if they are hindering the Southern Ocean’s capacity to pull carbon out of the atmosphere.
“There are questions about what’s going to happen if the winds continue to get stronger,” says paleoclimate scientist Krystyna Saunders of the Australian Nuclear Science and Technology Organisation in Sydney. “How much can the oceans actually absorb?”
Saunders and her colleagues are finding clues that over the past 12,000 years of Earth’s history, higher wind speeds and greater concentrations of carbon dioxide in the atmosphere have gone together. If this link between wind and CO₂ holds true today, it will lead to higher levels of the greenhouse gas in the atmosphere, which will speed up climate change, which could further intensify the westerlies.
There’s growing evidence that, at least in the winter months when the westerlies are at their most violent, some parts of the Southern Ocean are giving off more of the gas than was previously estimated. It has all the makings of a vicious cycle.
Earth’s expanding midriff
The westerlies are the result of a global atmospheric circulation system, starting with what is called the Hadley cell. The cell forms as warm, moist air near the equator rises into the upper atmosphere and then, as it cools and dries, much of it sinks down toward Earth at about 30 degrees latitude in each hemisphere. Lower in the atmosphere, this air moves back toward the equator, completing the cell. Some of this downward-moving mass of air escapes the Hadley cell and moves away from the equator, and is deflected by Earth’s rotation, creating the westerlies. Because the westerlies form at the edge of each hemisphere’s Hadley cell that is closest to the pole, the bigger the size of the cell, the more the winds move toward the pole.
Data collected by weather balloons, satellites and other means going back to 1960 show that Earth’s tropical belt has widened in the past few decades by a few degrees of latitude, effectively expanding the size of the Hadley cells. This is partly due to global warming, as predicted by climate models. At the same time, the westerlies have been pushed poleward. With less distance to cover as they circle a narrower part of the globe, the law of conservation of angular momentum dictates that the winds should increase in speed, much like an ice skater rotates faster when she draws in her arms. And measurements during the past three decades show that the westerlies have, indeed, intensified.
The westerly winds encircling the globe in both hemispheres between 30 and 60 degrees latitude are driven by two major forces. Global atmospheric circulation systems called Hadley cells are created by warm air rising near the equator, traveling toward the poles and then cooling and sinking back toward Earth near 30 degrees latitude in each hemisphere. The Coriolis force created by Earth’s rotation deflects some of that downward-moving air toward the east, which forms the westerlies.
As researchers were making sense of these changes to the westerlies, other scientists began using measurements of CO₂ levels in the atmosphere to determine whether the shifting, speedier westerlies are causing a drop in the amount of CO₂ being absorbed by the Southern Ocean. They had reason to suppose that this would happen.
Like any gas, carbon dioxide exerts a pressure that depends on the amount of the gas relative to other gases in a mixture. If this so-called partial pressure of carbon dioxide (pCO₂) of the atmosphere is greater than the pCO₂ of the ocean, then the ocean will absorb carbon from the atmosphere. Otherwise it will release it. And as the westerlies get stronger, the pCO₂ of the Southern Ocean could change, due to shifts in the pattern of ocean currents.
The westerlies drive the Southern Ocean current that circles Antarctica. The predominantly west-to-east current is further acted upon by a force generated by Earth’s rotation, known as the Coriolis force, which deflects moving objects to their right in the Northern Hemisphere and to their left in the Southern Hemisphere. The current circling Antarctica is deflected to its left, or northward, pushing the surface waters away from the pole. This sets the stage for water from deep in the Southern Ocean to move to the surface. Up to 80 percent of all the deep water that returns to the surface around the globe makes that return in the Southern Ocean. “It’s unique,” says climate scientist Joellen Russell of the University of Arizona in Tucson. “There's nothing like it in the world.”
This massive upwelling of the deeper, carbon-rich waters of the Southern Ocean increases the pCO₂ of the ocean surface, which decreases its capacity to act as a carbon sink, the argument goes. But is this what is actually happening?
Secrets of the deep
The Southern Ocean has been loath to reveal its workings. This is due to the complicating effects of local wind patterns and the fact that researchers are only just beginning to make direct measurements of the outgassing of CO₂ during peak winter months there. So far, the data paint a somewhat confusing, and concerning, picture.
These maps show the tracks of research vessels and other ships that measured pCO₂ at the surface of the Southern Ocean between 1980 and 2017. Each dot represents an area where at least one measurement was made, and the colors indicate the number of months during each decade that measurements were taken in each location. Even though the number of measurements has gone up dramatically in recent decades, the data are still quite sparse in space and time.
Previous estimates had shown that between 1981 and 2004, the Southern Ocean absorbed about 80 million fewer metric tons of carbon per year than expected. This was a cause for concern, given that the Southern Ocean is “the most important marine carbon sink on the planet,” says ocean biogeochemist Peter Landschützer of the Max Planck Institute for Meteorology in Hamburg, Germany.
So Landschützer and his colleagues took a closer look at a global database of pCO₂ values, created from observations of surface waters by instruments deployed in the sea or carried on research and commercial ships. Known as the Surface Ocean CO₂ Atlas (SOCAT) and established in 2007, it contains almost 26 million observations of global oceans and coastal seas from 1957 to 2019. Since data are sparser for the earlier decades than for recent years, Landschützer’s team filled in the gaps using other, less direct but more frequently measured parameters such as sea surface temperature and salinity and their well-understood relationship to pCO₂ of surface waters.
They estimated that the Southern Ocean had indeed taken in less CO₂ than expected from 1982 to 2001. The trend reversed in 2002, with the ocean acting as a more vigorous carbon sink all the way up to 2011. From 2011 to 2016, however, the Southern Ocean went back to being less effective in its carbon absorption. The team tried to find a link between the changes in the Southern Ocean’s ability to absorb CO₂ and the poleward-shifted westerlies. “We were quite surprised that we did not,” Landschützer says.
This called for an explanation, and the answer turned out to be complicated. Further analysis showed that the changing westerlies are, indeed, increasing the upwelling of carbon-rich deep waters near Antarctica. This effect, though, is being offset further north in the Southern Ocean by an increased carbon uptake in regions where the westerlies are blowing less vigorously than in the past. This would imply that there should be no overall change in the absorption of carbon. But Landschützer’s team found that the Southern Ocean’s ability to absorb carbon is increasing or decreasing over timescales of roughly a decade. The researchers put these flips down to the local wind patterns in different parts of the Southern Ocean known as the Atlantic, Pacific and Indian sectors. Winds there seem to change every decade, as evidenced by about 35 years of data, and those local alterations line up well with carbon absorption trends, Landschützer says.
All of the above analysis was based on data collected by ships plying the Southern Ocean mainly during summer. The data for the winter season was spotty; it is hard for ships to venture close to Antarctica at that time. So Landschützer’s team used the richer summer data and the sparse winter data to extrapolate and account for the winter months as best they could.
But in recent years, researchers have started using floats that are offering a new, broader glimpse of winter goings-on in the Southern Ocean. And the data are disconcerting. Oceanographer Alison Gray of the University of Washington and her colleagues used data collected by 35 of these autonomous floats, deployed by the Southern Ocean Carbon and Climate Observations and Modeling (SOCCOM) project. The floats, which had continuously monitored the top 2,000 meters of the Southern Ocean waters from May 1, 2014, to May 1, 2017, have sensors for a range of ocean surface parameters, and these can be used to calculate the pCO₂ of the surface waters. The analysis by Gray’s team showed that the Southern Ocean around Antarctica is outgassing significantly more CO₂ in the winter than Lanschützer’s methodology estimated.
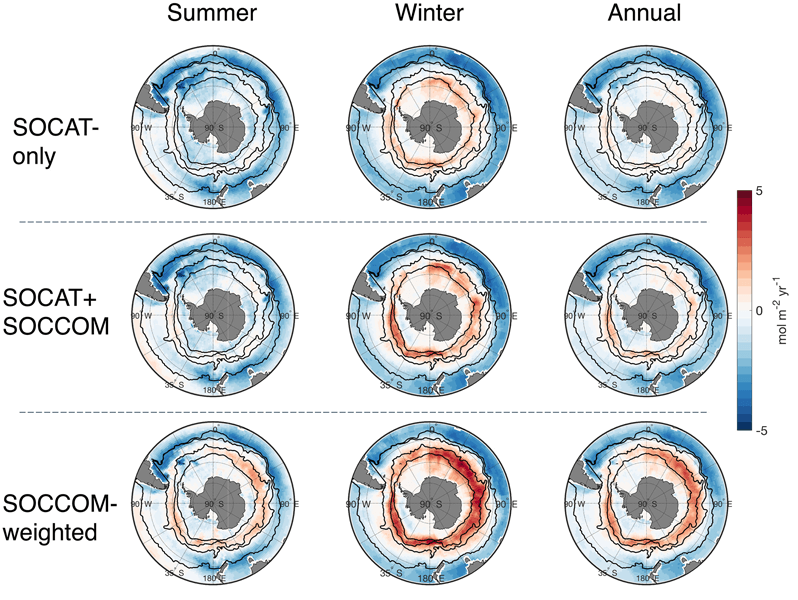
Data collected largely from ships crossing the Southern Ocean (top line) show strong uptake of carbon dioxide by the ocean — indicated by blue — in summer (left), and moderate outgassing of carbon dioxide from the ocean —shown in red — during the winter (center). Data from the surface float system called SOCCOM (bottom line) show much stronger outgassing during the winter. The truth is likely somewhere in between, as shown by combining both data sets (middle line).
CREDIT: S.M. BUSHINSKY ET AL / AGU GLOBAL BIOGEOCHEMICAL CYCLES 2019
To see if they could resolve the discrepancy, Landschützer and colleagues combined the data sets and found that “the truth lies somewhere in the middle,” he says: The carbon uptake is not as low as the direct float measurements suggest, but neither is it as high as estimated by the ship-only data.
While more work will help climate scientists understand exactly what’s happening in the Southern Ocean, for the University of Arizona’s Russell, the direct winter data from the SOCCOM floats is already raising alarms. “In the deep winter, when the winds are howling the strongest and the deepest layers are being stirred to the surface, that’s when we're tapping those older carbon waters,” she says. “And that's what's worrisome.”
A salty log
The deep waters are carbon-rich because of the microscopic marine algae called phytoplankton that live in the surface waters. The phytoplankton photosynthesize the CO₂ in the water into organic molecules to build their bodies. When they die, they sink to the ocean floor, sequestering the carbon for centuries. Some phytoplankton are consumed by larger organisms called zooplankton, which in turn are eaten by even bigger creatures whose carbon-rich fecal pellets and dead bodies can also end up on the ocean floor, burying ever more carbon in the deep waters.
The evidence from the SOCCOM floats suggests that it’s these deep waters that are upwelling during the Antarctic winters. “It's the old rotted water that normally takes hundreds of years to circulate out of the deep,” Russell says.
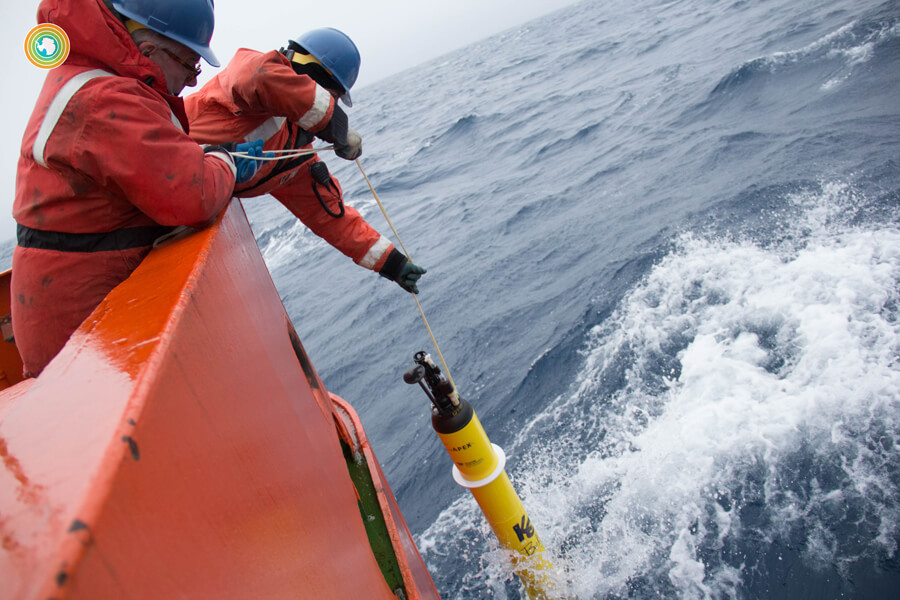
An autonomous float is lowered into the waters of Southern Ocean. Part of the SOCCOM project, floats like this measure a variety of parameters that allow scientists to determine whether the waters are absorbing or releasing carbon dioxide.
CREDIT: SOCCOM PROJECT
Scientists surmise that intensifying westerlies are bringing more of this carbon-rich deep water to the surface — but they need more evidence. One way to confirm that speedier westerlies have an impact on Southern Ocean outgassing, and thus on the levels of atmospheric CO₂, is to look at past climate evidence. Krystyna Saunders and her colleagues decided to look for some of that evidence on subantarctic Macquarie Island, which lies about 1,500 kilometers from Tasmania, midway between Australia and Antarctica. At latitude degrees 54S, it’s right in the main band of the westerlies.
Getting to Macquarie Island is no small feat, even on the Aurora Australis, a 311-foot-long icebreaker. Despite its size, the ship can be tossed around by massive swells, the kind Shackleton wrote about. “You can be lying down in your bunk, rolling from side to side and head to toe as the ship swirls around,” Saunders says. “If you get seasick like me, it’s quite horrible.”
On Macquarie Island, Saunders and her colleagues capitalized on a fortuitous natural record that provides clues to how strongly the winds around the island have blown in the past. That record lies at the bottom of Emerald Lake, a body of freshwater on the island’s western edge. It’s exposed to the westerlies and thus to salty ocean spray blown in by the winds: The greater the wind speeds, the higher the salinity of the lake. The salinity in turn affects which species of diatoms, types of algae, can thrive in the lake.
When they die, diatoms sink to the bottom of the lake and are buried in sediments on the lakebed, creating a layered chronicle of the salinity of the lake — and by proxy the speed of the winds. Saunders’ team extracted sediment cores from the lakebed, focusing on layers that had accumulated during the Holocene, the present epoch in Earth’s history that began about 11,700 years ago at the end of the last ice age.
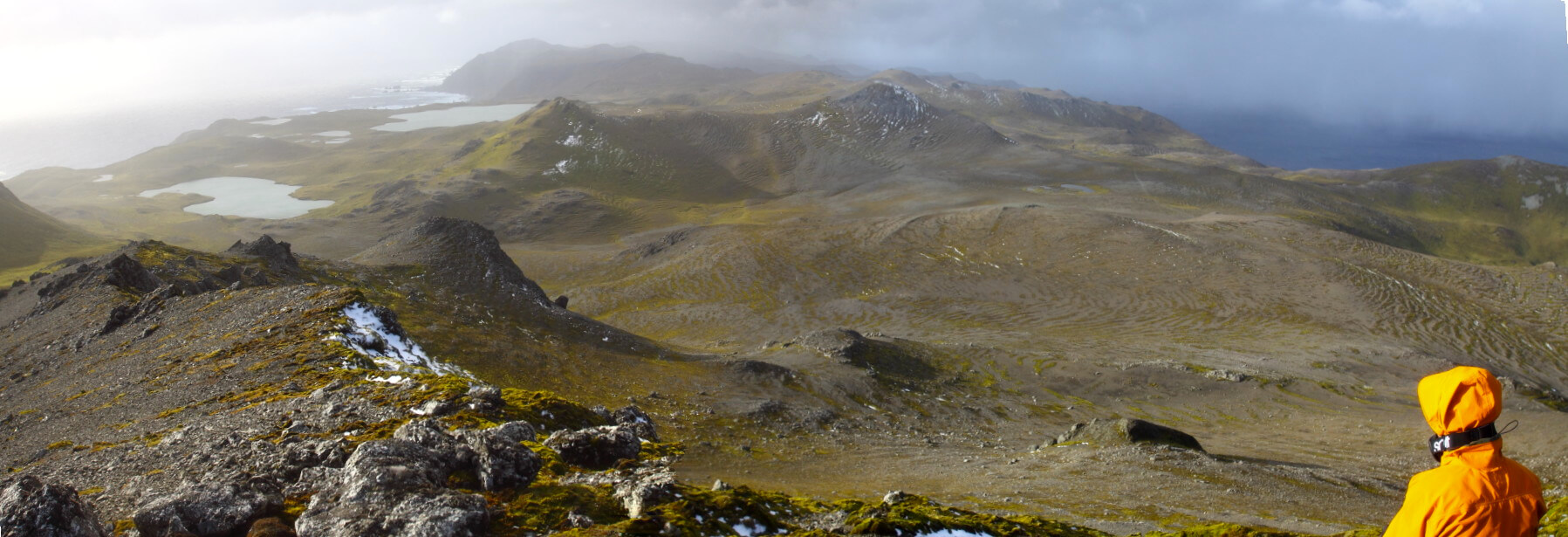
Lakes on remote Macquarie Island, which lies in the main band of the westerlies, midway between Australia and Antarctica. Scientists have taken sediment core samples from the bed of Macquarie’s Emerald Lake to reconstruct a record of past wind speeds.
CREDIT: KRYSTYNA SAUNDERS
Having inferred the speeds of westerlies over the course of the Holocene, they then looked at data from ice cores drilled in Antarctica to get a direct measure of the amount of CO₂ in the atmosphere over that same time period. They found a clear correlation. “If you look at periods when the winds were above their average strengths, and periods when carbon dioxide was above its average concentration, they overlap,” Saunders says.
This has implications for our understanding of the effect the westerlies have on the Southern Ocean’s capacity to absorb carbon. Saunders’ study supports the idea that as the westerlies speed up, the Southern Ocean will outgas more CO₂ — as shown by Gray’s report on wintertime data collected by the SOCCOM floats — and goes against the analysis of 35 years of ship-based data by Landschützer’s team. Only more studies, along the lines of Landschützer’s attempt to reconcile the various datasets, will help resolve this debate.
Awakening a beast
There’s one more factor that could feed into the vicious cycle that scientists fear may already be underway in the Southern Ocean. Changes in the extensive Atlantic Ocean circulation system that includes the Gulf Stream may also be exacerbating the warming of the Southern Ocean, which in turn could be adding to the poleward shift and intensification of the westerlies.
This circulation system, called the Atlantic meridional overturning circulation, or AMOC, brings warm, salty surface water to the north, where it cools and sinks and then heads back to the Southern Hemisphere through deeper ocean layers. Scientists have found evidence that the AMOC has been slowing down, particularly in the last 150 years compared to the preceding 1,500 years. One indication of this is that particles being deposited on the ocean floor have been getting smaller and smaller: Fast-flowing water can whisk away smaller particles, allowing only the larger particles to settle, whereas slower water allows smaller and smaller particles to drop to the floor.
This video taken from a Royal New Zealand Navy vessel offers a taste of how foul the weathers in the Southern Ocean can get.
CREDIT: ALEX CROUCHER
Some of the AMOC’s slowdown can be attributed to one of the consequences of global warming: an increasing supply of glacial meltwater into the North Atlantic. This fresh water reduces the salinity, and hence the density, of the surface waters. The formation of dense, salty, cold water is essential for it to sink down to the deep ocean in the North, from where it makes its return to the Southern Hemisphere. This process is being disrupted by climate change.
Since 2004, a project called RAPID has deployed moorings across the breadth and depth of the Atlantic at latitude degrees 26N (stretching from Florida to Morocco), to directly measure changes in the velocity of the AMOC. The measurements show that the current is indeed slowing down. But because of inherent decade-to-decade variations in the climate system, 15 years’ worth of direct measurements isn’t enough to establish a trend.
So Levke Caesar, then with the Potsdam Institute for Climate Impact Research in Germany, and her colleagues extended this record back in time by using actual measurements of sea surface temperatures from 1870 to 2016, combined with a high-resolution climate model to reconstruct the strength of the AMOC over the past 150 years or so. Their work shows that the circulation system’s strength declined to a minimum around 1990, then grew until the early 2000s, and then started declining again. Other scientists have found similar results. As long as greenhouse gas emissions continue to rise, “all climate models predict a slowdown of the AMOC,” Caesar says.
Because the AMOC transports heat from the south to the north, a slowdown effectively cools the Northern Hemisphere and heats up the Southern Hemisphere. This warming also intensifies the westerlies and shifts them toward the South Pole, leading to a greater vertical churning of the Southern Ocean, which may contribute to global warming by weakening the ocean’s ability to absorb CO₂. Since the likely result is even more glacial meltwater flowing into the North Atlantic, this could further weaken the AMOC. It has all the makings of a system that’s slipping out of control.
A similar runaway process is thought to have ended the last ice age, raising CO₂ levels from about 180 to 240 parts per million. This rise happened from about 17,500 years ago to 11,700 years ago, causing the deglaciation that made Earth more habitable. Atmospheric CO₂ levels are now past a whopping 410 ppm due to human activity, and rising. In a mere 250 years, we have increased CO₂ levels by more than the amount that melted an icy planet over thousands of years.
The winds are howling again over the Southern Ocean and are dangerously close to awakening a beast that, until now, has been helping us by absorbing copious amounts of carbon. But even the Southern Ocean is unlikely to cooperate forever.
Editor's note: An earlier version of the map showing how the westerlies are made had incorrect placement of Earth’s geography. The map has been redrawn and was updated on April 1, 2020.