Listen on: Amazon Music | Apple Podcasts | Deezer | Google Podcasts | LibSyn | Player.FM | SoundCloud | Spotify | Stitcher
This is Episode 4 of Season 3 of the Knowable Magazine podcast. Listen to Episode 1 (Scientists warned about climate change in 1965. Nothing was done.), Episode 2 (The fossil that launched a dinosaur revolution) and Episode 3 (How the placebo effect went mainstream).
Transcript
Adam Levy: What does quantum physics tell us about the nature of reality? Could distant particles hold an influence over each other? And is the universe really spooky? I’m Adam Levy, and this is Knowable.
This season we’re investigating just how and when ideas in science develop. Each episode looks at how one text changed the way we think, and in this episode we are looking at one text — in fact, one phrase — that’s stayed with physics for some 80 years: “spooky action at a distance.”
This is Einstein’s memorable choice of words for something that he felt was deeply wrong with the emerging ideas of quantum physics. In this episode, we’ll take a look at what Einstein found too spooky to accept, and the Northern Irish physicist John Stewart Bell, who devised a way to finally test the universe and find out whether Einstein’s skepticism was well-founded.
But before we get to that, we need to talk about the revolution that was taking place in physics in the early 20th century. It became increasingly clear that the universe didn’t work how physicists had classically described it.
Classical physics describes the world with deterministic laws which govern waves such as light and particles like electrons, but this couldn’t describe a host of phenomena that experiments had unveiled. And so this classical view of the universe was gradually being replaced by quantum mechanics, which described bizarre properties of the very small.
Nicolas Gisin: At the beginning, it was really what people call wave-particle duality.
Adam Levy: This is Nicolas Gisin, at the University of Geneva, who has worked his entire career on applied physics and quantum physics, having spent decades reflecting on quantum physics applications to communication.
OK, but wave-particle duality?
Nicolas Gisin: So you have a particle, an electron, or an atom, and all these particles will sometimes behave like a particle, so like a little billiard ball, but sometimes [they] will behave like a wave. And so this dual nature of these particles was really what kicked off everything, all quantum science, let’s say. Until the ’60s that was, and probably even later, that was really the main concern.
Adam Levy: The notion that the building blocks of the universe weren’t either waves or particles, but somehow behaved as both, turned physics on its head. But while this may have been the main concern, it was far from the only concern about the strange implications of quantum physics.
Quantum physics predicts that particles can become entangled. This suggests that two or more particles can be brought together and become fundamentally linked so that even when they’re separated, even by great distances, it’s no longer possible to describe them independently of each other. And observing one apparently affects observations of the other.
Nicolas Gisin: Entanglement has the following implication: If I now measure or do something on the first particle, somehow the second one gets affected, or the second one “shivers.”
Adam Levy: Such an idea challenged the core of Einstein’s idea of the universe, that messages could only travel through the universe at the speed of light or slower, and so no object could instantaneously influence an object separated from it. But here was quantum physics suggesting that measuring one entangled particle could cause immediate effects, or shivers, on the other.
Nicolas Gisin: Yeah. So Einstein, like many people at that time, realized that if now you do measure on one side, it has a kind of effect on the other one. One of these particles could be, let’s say, in Geneva where I’m sitting, and the other particle could be, I don’t know, in the US somewhere at a far distance, and nevertheless, these particles have this property of entanglement so that you cannot describe them just separately. And Einstein just couldn’t believe it, so he concluded entanglement is impossible.
Adam Levy: Einstein actually in 1947 wrote a letter to Max Born in which he nicknamed what we’re talking about, this non-locality, a “spooky action at a distance.” This phrase is still used to this day, but at the time, was Einstein kind of out there on his own in rebelling against these ideas, or was there a real active discussion among physicists at large?
Nicolas Gisin: Yeah. So I think Einstein several times was very accurate and inventive in coming [up] with sentences like, “God does not play dice,” or, “spooky action at a distance.” So this “spooky action at a distance” really reflects this idea that you touch the particle here in Geneva and the one in the US is shivering. So there is a kind of action at a distance. And Einstein said, “This is a spooky, it cannot really be a real one. It has to be a spooky action.”
At that time, I would say, surprisingly, physicists didn’t pay much attention to that … the very, very vast majority of physicists at that time did not at all pay attention to these questions and issues. It was also at that time impossible to turn it into, let’s say, an experiment. And some people were even thinking that this is really pure philosophy and has no physical consequences. We’ll see later that these people were completely wrong, but that was the dominant view.
Adam Levy: It wasn’t until actually after Einstein’s death, [that] in 1964 John Stewart Bell proposed a test that could potentially settle the matter. What’s the essence of this test, which we now call the Bell test?
Nicolas Gisin: So imagine now that you have your two particles, they are maximally entangled, very highly entangled, and for the sake of the explanation, think of them as coins. And so you get two possible results. If you toss your coin, it can be heads or tails. Now if you throw them the same way on both sides, the two coins, they will always both end up either both heads or both tails.
Adam Levy: This is the spookiness that quantum physics predicts, and Einstein found so unbelievable. The idea that if two particles are entangled and you separate them, you’ll get the same result if you measure a property of both of them, like being guaranteed to get the same result when tossing two separate coins. It’s as if both particles instantaneously agree to both show heads, for example.
But there could be a non-spooky explanation. What if, before you separated them, the particles made a secret plan deciding what they’d show for the coin toss. Then there’d be no quantum entanglement, just the particles putting their plans into action.
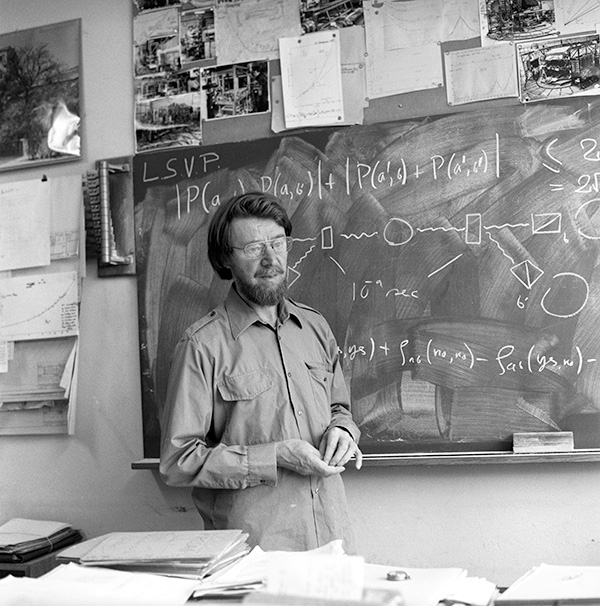
Proof of quantum entanglement has come in a handful of experiments completed in the half century since John Stewart Bell first devised a way to test just how spooky it is. Bell, a theoretical physicist, is shown here at CERN in a 1982 photo.
CREDIT: CERN
Nicolas Gisin: Also, this genius idea of John Bell was to change a little bit the way of tossing the coins. So let’s say now one coin is tossed the usual way, but the other one is tossed a little bit differently.
Adam Levy: In other words, what if we measure the two particles but in subtly different ways? Will they both then still agree with each other, metaphorically both showing heads, for example?
John Stewart Bell, a theoretical particle physicist who found time to work on quantum theory on the side, realized that these slightly different coin tosses could settle the matter, deciding whether the particles really were entangled or if Einstein was right to reject this quantum spookiness. If Einstein was indeed correct that there was no entanglement, and instead the particles had agreed a secret plan in advance, there was a limit, an upper bound, to how often the two particles could have the same result if physicists repeated the coin toss experiment many times.
Nicolas Gisin: While with quantum mechanics, you can violate that bound, so the bound is what we call a Bell inequality.
Adam Levy: So if quantum physics were right, and the particles really were entangled and there really was “spooky action at a distance” going on, when repeating the experiment, you’d end up with both particles showing heads together or both showing tails together more often.
That’s because the particles really would be linked, and so really able to instantaneously influence the result of each other’s coin toss experiment. In other words, this Bell test was a way to test which was right: the quantum prediction of entanglement, or Einstein and his skepticism of such bizarre behavior.
Nicolas Gisin: So this is really what Bell proposed, and turned this entire discussion into a potential experiment.
Adam Levy: Now, Bell didn’t actually carry out his own test, he proposed it. But when did someone actually come along and make an experiment that could apparently show where the particles were indeed entangled in this spooky way?
Nicolas Gisin: Yeah. So John Bell was a theorist and he didn’t know how to do the experiment. We had to wait almost 10 years, into the ’70s, to have the first experimental demonstration of achieving this large probability of identical results when you measure slightly different measurements, and this was done by John Clauser.
Adam Levy: John Clauser’s approach to the Bell test was to manipulate calcium atoms to create two apparently entangled photons, particles of light. These photons could then be tested with those metaphorical, slightly different, coin tosses, which in this case meant measuring the direction of the light’s polarization, which direction the wave was wiggling. Clauser could then see how often the two photons produced the same result. And indeed, the experiment showed a violation of the Bell inequality, indicating that the photons really were entangled. Einstein’s assumption appeared to be wrong.
Nicolas Gisin: And this was an absolutely marvelous result. The problem of John Clauser.... Actually, he had two problems.
First, there was another guy at the other side of the American coast who also did the experiment just a bit after John Clauser, but he found a different result. So obviously one of them was wrong, but how do you know which one was wrong?
Moreover, in the ’70s it was still believed that all that is not serious physics, and John Clauser actually was never promoted in any university to any professorship. So his career was actually damaged, strongly damaged, by having been the first to perform a Bell test.
And then came another experiment, but that was now already in the ’80s. So each time, you see, it’s almost 10 years, one after the other.
And that third experiment was carried out in Paris, in France, by Alain Aspect and his coworkers. And they made an experiment — much higher quality, a much better experiment, also it took longer. And they kind of settled the question in favor of quantum mechanics. Hence, entanglement is certainly a real feature of nature and not only a theory.
Adam Levy: So settled in favor of quantum mechanics and against Einstein’s disbelief. These experiments suggested that, “spooky action at a distance,” was a real phenomenon. But I understand that that wasn’t case closed, that there still were some loose ends that needed to be tidied up, which are often referred to as loopholes.
In particular, the idea that instead of having instantaneous influence on each other’s coin tosses, the particles could somehow have a trick up their sleeve allowing them to still make a plan in advance and somehow cheat the test. Physicists call this possible particle trickery local variables. Can you explain efforts to close such loopholes and determine for sure that entanglement is a real feature of nature?
Nicolas Gisin: The major loophole in Alain Aspect’s experiment was that to carry out this experiment you need to produce these photon pairs.
OK, you don’t send them to Geneva and to the US, but you send them to the two ends of a big laboratory which is about 10 meters long. So the photons were 10 meters apart, and then you have to measure them.
However, in the real experiment, when you perform these measurements, the most frequent outcome is that you don’t get any results, just because the photon got lost on its way, or also the detectors are not a hundred percent efficient, so the detector very often just doesn’t see the photon. A photon is — it’s very easy to lose it or not to detect it. So indeed you could now imagine that these hypothetical local variables would decide when the photon gets detected and when the photon would not get detected. You could explain the results of the experiments, although everything would be guided and driven by local variables.
So this was something that still had to be tested. And this additional test came actually only in 2015, so almost 30 years after Alain Aspect. It took a long time to have good enough single-photon detectors.
Adam Levy: And this test effectively used a combination of photons and electrons to get around this problem of detection, also allowing the measurements to be carried out on an entangled pair separated by over a kilometer. And since then, there’ve been a host of other Bell tests closing other types of loopholes, and pushing the limits of the field, using everything from satellites to computer games.
But isn’t all this checking for loopholes a bit paranoid of physicists to think that somehow the particles are conspiring to trick the test, and make us think they’re behaving in a spooky way?
Nicolas Gisin: It is certainly paranoiac. However, the result of a Bell inequality violation means that this feature of quantum theory is not just a feature of a theory, it’s a feature of nature. So it changes the worldview that physics presents us. And so this is such a major change, it’s such a conceptual revolution, that it makes sense to pay attention, even to this hypothetical local variable that would decide when the photon should get detected or not.
So I think it makes sense really to go down to the bottom, remembering that actually physics is just an experimental science and it’s not enough just to contemplate the theory, you have also to really do the experiment. So I think it made a lot of sense, but I agree that almost no one, I think also none of the experimentalists, none of them believed that they would falsify quantum mechanics. Nevertheless, it made sense to do it.
Adam Levy: Now, has this all been theoretical, or has learning about the quantum world in this way and how to do these experiments led to any applications for the more mundane everyday world?
Nicolas Gisin: Indeed. So when it started with Einstein, that was kind of purely philosophy, then John Bell turned it into a possible experiment, John Clauser made the first experiment, Alain Aspect the first, let’s say, really conclusive experiment. And then suddenly in the ’90s — so [in the] 1990s, people realized that actually this kind of very abstract thinking had practical consequences, because indeed, if you get between the two particles always, or almost always, the same result, and this result is random, so you get randomness at a distance. And nonlocal randomness is very close to being just a cryptographic key.
Cryptographic key, you can think of it as a password. What is a password? It has to be the same on both sides, let’s say on your side and Amazon, or your bank, whatever, whoever you want to talk to in a confidential way. And it has to be random.
So here we have it already. Moreover, something that we also now understand in physics, with this theory of entanglement, is that if two particles are very highly entangled, then they cannot be entangled with anything else. They cannot be entangled with a third particle.
And consequently, that also guarantees the confidentiality. So if you and the bank are entangled, you can obtain the same random password on both sides. Moreover, you can be sure that there is no one else who has a copy of that password, and that’s then just exactly what you want. And so cryptography is actually very close in spirit to the violation of a Bell inequality.
But this was a complete revolution, suddenly realizing that these strange quantum correlations, Bell inequalities, potential or absence of local variables, actually are cryptographic keys. So they are very useful in an information-based society like ours.
Adam Levy: So the 2022 Nobel Prize for Physics was actually awarded for work to realize the Bell test. What is the significance of this acknowledgement of this work with this honor?
Nicolas Gisin: Well, this Nobel Prize of 2022, I think is not only a recognition to the three laureates [John Clauser, Alain Aspect and Anton Zeilinger], it is also a recognition that this entire field, which was so much dismissed during so many decades, is now recognized at the highest level. I have to say that the Nobel Committee made a huge mistake not to give a prize, the Nobel Prize, to John Bell. He should have got it, but he passed away too early, or let’s say that the Nobel Committee was too slow, or whatever.
So I’m personally very happy with this prize, because again, of course it goes to these three individuals, but beyond these three individuals it really recognizes a field that was criticized so heavily. And the best illustration is John Clauser, who was the first, who did a good Bell test and never could even get a position in any university. So much of these activities were dismissed, until 50 years later he got the Nobel Prize. So that’s really remarkable, I don’t know how often it happened that any field in science gets dismissed for such a long time, for really many decades.
When I started myself, I mean, it was impossible to work in this field except, let’s say, after 9 o’clock in the evening — you could not make a living out of that. And now the Nobel Committee recognizes it. That’s nice.
Adam Levy: Is it now case closed? Can we now say with certainty that the universe really is spooky and that there are no loopholes, no conspiring particles to trick us in these Bell tests?
Nicolas Gisin: Yes, except that I wouldn’t use the word “spooky,” I think that there’s nothing spooky here, but on the contrary, this is very, very real. I mean, this is now done routinely in the labs, you have even students doing that, those exercises in the lab. So it is something extremely well-established.
Adam Levy: How do you feel about how far we’ve come in our understanding of quantum behavior in the last, well, I guess in the last hundred years, really?
Nicolas Gisin: I would say in the last 30 years essentially, because for me it really started, the understanding started really with this understanding that these nonlocal quantum correlations produces naturally cryptographic keys, so something useful. And you can see the change.
Initially everything was about wave-particle duality, and nowadays almost no one talks about wave-particle duality. People are talking about entanglement. Entanglement is really what changes, what makes quantum mechanics so particular. While today, I think there’s no paper in the field, there is no book in the field, there is no lecture that doesn’t mention entanglement. So entanglement is at last understood as being the essence of quantum mechanics.
Adam Levy: And indeed, today, entanglement, this, “action at a distance,” that Einstein found so inconceivable is at the heart of many of our quantum technologies, whether that’s new forms of encryption, ensuring secret and safe communication between you and your bank, or the long-running quest to build a quantum computer with real-world applications. And to this day, it’s common to find physicists and journalists alike referring to quantum entanglement as quantum spookiness.
It took a long time for physicists to reflect on Einstein’s complaints about quantum physics, and even longer to prove him wrong, and show that entanglement is a fundamental way of how our universe works, with some kind of synchrony between separated particles. But today’s physicists are wasting no time at all putting “spooky action at a distance” into action.
Thank you for listening to this episode of the Knowable Magazine podcast. To learn more, you can find a copy of the transcript, along with links to the related papers at knowablemagazine.org/podcast. You’ll also find a link to sign up for the Knowable Magazine newsletter. We hope that you’ll subscribe so that you can always stay in the know.
This is the last episode of this season, but check out our previous seasons to learn about everything from understanding dreams to the hunt for planets beyond our solar system. And make sure you’re subscribed so you don’t miss what we’ve got coming next.
If you enjoyed listening to this episode, you can help others find it and enjoy it too by sharing it with friends and family or by leaving us a five-star review.
This show is produced by Knowable Magazine, a journalistic publication that seeks to make scientific knowledge accessible to all. Knowable is published by Annual Reviews, a nonprofit publisher dedicated to synthesizing and integrating knowledge for the progress of science and the benefit of society.
This episode was written and produced by me, Adam Levy, with editorial contributions from Eva Emerson and Rosie Mestel. Thank you to my cohost, Charlotte Stoddart. And a special thank you to Nicolas Gisin for speaking with us.
For more smart stories about sound science, go to knowablemagazine.org.
I’m Adam Levy, and this has been Knowable.