It’s not the most common cause of blindness. That dubious distinction goes to diabetes, followed by maladies such as cataracts, glaucoma and macular degeneration. But for the one person in 4,000 afflicted with the genetic disorder retinitis pigmentosa, it is devastating — a slow, inexorable death of the rod and cone cells that serve as the eye’s light detectors. Night vision is the first to go, usually in childhood. Then comes the gradual loss of peripheral vision, even in daylight. By age 40 or so, people with the condition are typically left with only a small, central patch of vision, as if they are looking at the world through a straw.
The good news is that this particular form of blindness leaves an opening for technology. Two types of artificial retinas have already been approved for human use. And new work suggests a way for those retinas to one day get much better.
The key point is that retinitis pigmentosa targets the rod and cone cells almost exclusively. The disease does minimal damage to the retina’s many other neurons, which process signals from the rods and cones and convey the results to the optic nerve. So in principle, fixing vision is just a matter of going in through the very back of the eye, where the ravaged rods and cones originally formed a layer just 100 micrometers thick, and replacing them with a device that will generate electrical pulses in response to light. Pulses from various points on the device can then communicate with the retina’s surviving neurons in a natural way.
Existing retinal prostheses require silicon or metal implants that are comparatively thick and completely rigid, a combination that the sensitive retinal tissue does not like at all, says physicist Guglielmo Lanzani of the Italian Institute of Technology (IIT) in Milan. “Over time,” he says, “inflammation is followed by fibrosis” — scarring, which can reduce the artificial retina’s already limited effectiveness.
So instead, as Lanzani and his IIT colleagues explain in the recent Annual Review of Physical Chemistry, their group is investigating a different kind of retinal prosthesis made from semiconductive polymers, a class of carbon-based plastics that can conduct electricity in much the same way that silicon microchips do.
Photoreceptor cells at the back of the retina allow the eye to detect light. Rods provide vision in low-light; cones allow us to see in full-color and fine detail in bright light. Both may be damaged in the inherited disease retinitis pigmentosa, while retinal neurons remain intact. Replacing damaged rod and cone cells with an artificial implant can help restore some vision.
These polymers are best known for their use in some types of organic light-emitting diode (OLED) displays, the richly colored screens found in millions of smartphones. But the materials also show promise for a new generation of cheap, flexible, lightweight solar cells. And they show even more promise as soft, flexible bioelectronic interfaces to living tissue — “one of the emerging and very exciting applications of organic semiconductors,” says Carlos Silva, a physicist at Georgia Tech in Atlanta. These applications include drug delivery and biosensors.
Because semiconductive polymers bend and flex like natural tissues, Lanzani says, “they are biocompatible.” In tests in the lab and in animals, the polymer retina seems to coexist with them quite happily, with no adverse reactions at all.
Just as important, adds IIT neuroscientist Fabio Benfenati, semiconducting polymers can get the physiology right. When light hits the polymer sheet, he says, it triggers a localized pulse of electrical activity about 80 to 100 micrometers across. Because this is roughly comparable to the spacing of rod and cone cells outside the densely packed fovea, where the eye’s visual acuity is the highest, the polymer prosthesis would allow a resolution akin to a person’s natural peripheral vision.
And because the sheet can be engineered to deliver its electrical pulses as a flow of ions, it can pass signals to the surviving retinal neurons in a way that they recognize: Ion flows are neurons’ native language. “Even though the mechanism is probably different from what is occurring in nature,” Benfenati says, “what we do is very biomimetic.”
When implanted into the damaged retinas of rats, the semiconductive polymer P3HT (shown in cross-section in pink atop two other materials in this falsely colored scanning electron microscope image) is positioned near to working bipolar cells. Rats with the implants recovered their ability to sense light.
CREDIT: H. KOLB ET AL / WEBVISION: THE ORGANIZATION OF THE RETINA AND VISUAL SYSTEM 2012
A light-sensitive polymer
Carbon-based polymers such as rubber, nylon and polyester are usually thought of as insulators, preventing electricity from flowing out of wires or other metal parts. But in the 1970s, chemists established that certain polymers could conduct electricity quite well — and better still, could function as semiconductors like silicon. By 2000, when work in this area won the Nobel Prize in chemistry, the field was flourishing.
Today, there are a number of semiconducting polymers that might be suitable for an artificial retina, but the IIT group has focused on P3HT, short for poly(3-hexylthiophene-2,5-diyl), a material widely used in photovoltaic cells. In 2007, recalls Lanzani, as part of an effort to develop a more accurate device for measuring colors, he and his physicist colleagues showed that P3HT could be engineered to respond to light in much the same way as green-sensitive human cone cells. And shortly thereafter, he says, “I heard about people building an artificial eye for robotics, including a retina-like detector, and I thought, ‘The best place for a retina is in the eye — the real eye!’”
This artificial retina idea became concrete when he met Benfenati at the coffee machine during an institute meeting. “Fabio was excited,” says Lanzani — and as a neuroscientist, he knew how to work with living neurons.
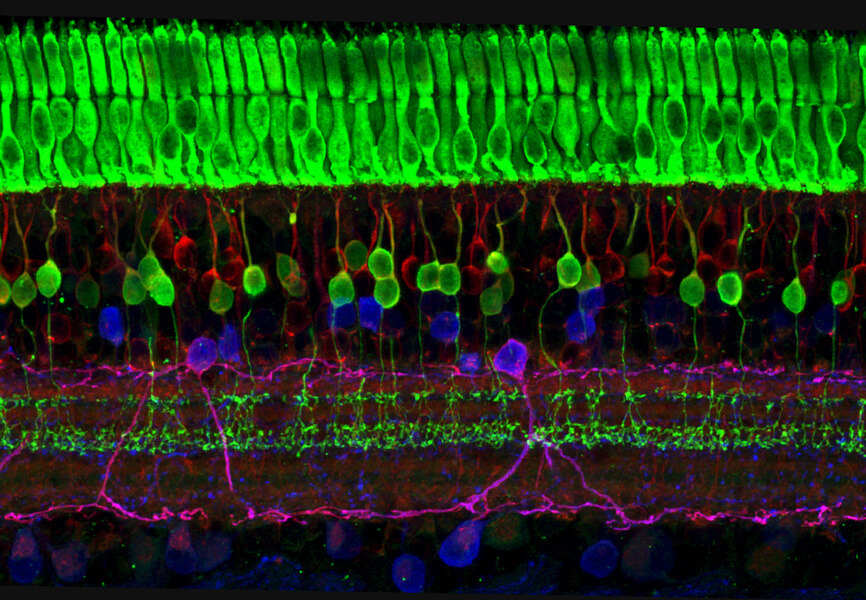
A healthy retina is made up of many layers of nerve cells that help convert light into energy impulses that are sent deeper into the brain. The light-sensing rod and cone cells are shown in green on top; the other cell types relay information from rods and cones into the optic nerve. An artificial retina can detect light and then send an impulse to adjacent nerve cells.
CREDIT: WEI LI, NATIONAL EYE INSTITUTE, NATIONAL INSTITUTES OF HEALTH
Joining forces, their teams showed in 2011 that neurons cultivated on a film of P3HT would indeed make connections with the polymer. What’s more, the neurons responded to electrical impulses from the polymer in the same way they would to nerve impulses from a rod or cone cell. Then in 2013 they showed that retinas taken from a strain of rat with dysfunctional rod and cone cells would connect to the polymer in the same way, and would have a similar response to impulses. That was a confidence-booster, says Benfenati. “It’s one thing to have a cell growing onto a surface and getting a very tight contact,” he says, “and another thing just to put preformed tissue in contact.”
Independently, as it happens, K.S. Narayan and his team at the Jawaharlal Nehru Centre for Advanced Scientific Research in Bangalore, India, were getting similar results using a polymer blended with P3HT. But since then, the two teams’ paths have diverged somewhat. Narayan’s group has been carrying out laboratory studies with photoreceptor-free retinas obtained from chick embryos to get a very precise understanding of how the polymer and the retinal neurons interact. “We are interested in biophysics,” he says — “how neurons get excited when we introduce these artificial polymers to replace the receptors.”
Lanzani and Benfenati, in the meantime, have moved on to testing their polymer prosthesis in the retinas of living animals. A crucial factor in this work has been their partnership with Grazia Pertile, says Benfenati. Not only is she head of the ophthalmology department at the Sacrocuore Hospital in Verona, Italy, he says, and one of the most skilled retinal surgeons in Europe, “she is very interested in basic research, as well.”
In 2017, Pertile and colleagues succeeded in implanting a full prosthesis in the eyes of living rats from a strain that has a genetic defect analogous to retinitis pigmentosa. After a month’s healing time, the pupils of these once-blind rats were contracting in response to light exactly like those of healthy rats, and their once-dormant visual cortex was abuzz with renewed activity. It’s impossible to know what the rats were actually experiencing, but they showed every sign of being able to see again.
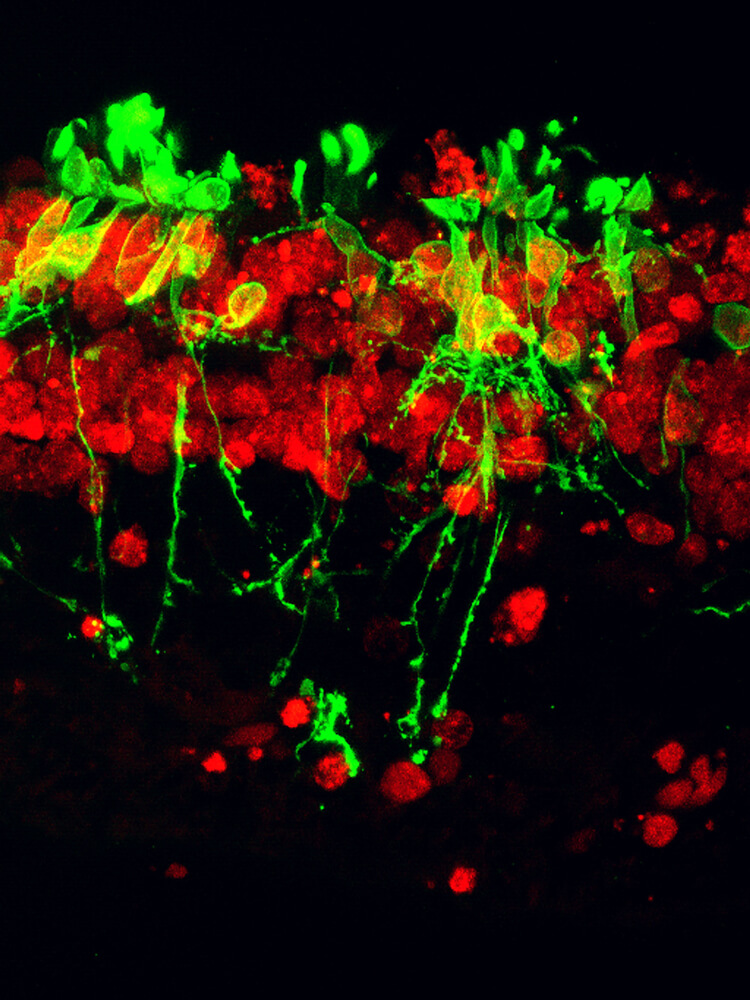
Light-sensing rod and cone cells (green) usually form an orderly, dense layer in the back of the retina. But in retinitis pigmentosa, first the rod and then the cone cells break down and the structure degenerates, leading to problems with night vision and eventually profound vision loss and even blindness.
CREDIT: ROBERT FARISS AND ANN MILAM, NATIONAL EYE INSTITUTE, NIH
The team has now embarked on the multiyear road toward human experimentation. To prepare the way, Pertile has been developing techniques to implant the polymer retinal prosthesis in pigs, whose eyes are similar to people’s in both size and visual acuity, while others on the team have been refining the prosthesis itself.
“We’re taking the time,” says Lanzani, “in order to have the best architecture before going to humans.”