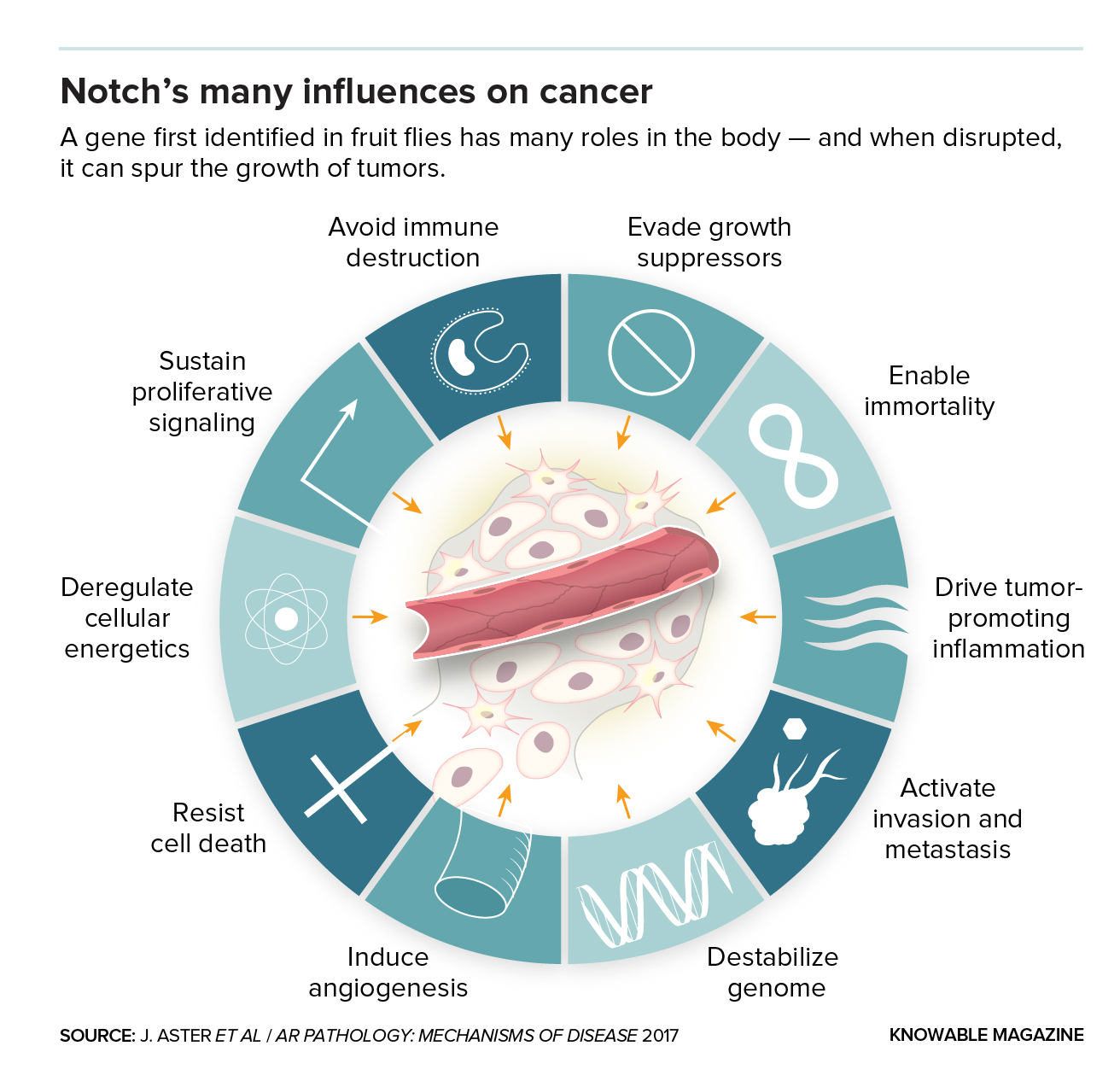
The wheel you see above symbolizes much suffering — the image portrays the many ways in which a single protein may cause, or contribute to, a laundry list of human cancers. The protein is called Notch, and we couldn’t live without it. But when it’s in overdrive (or in underdrive, for that matter) it may wreak havoc. Today’s growing understanding of Notch’s myriad jobs, and the dark results that follow when Notch goes rogue, is spurring new approaches to treating cancer and an often-fatal side effect of transplants called graft-versus-host disease.
Notch proteins, and the biochemical pathways they control, are ancient: All multicelled animals have them. In fact, the gene that carries instructions for making Notch was first identified in the fruit fly Drosophila melanogaster (the original mutation, found in 1917 by pioneering geneticist Thomas Hunt Morgan, caused a ‘notch’ on a fly’s wing). All over animal bodies, Notch transmits signals from the outside to the inside of cells, nudging them to become one type of cell or another. And when Notch is absent in developing embryos, those embryos soon die.
Here are the nuts and bolts of how Notch works: The protein straddles the membranes of cells, with one end poking toward the outside environment and the other poking into the cell’s interior. When an activating molecule docks onto the outside end, the inside part of Notch is snipped off. The snipped-off bit then travels to the cell nucleus, where it turns on scores of genes — different ones depending on the cell type.
That puts all kinds of processes into play: Nerve cells begin to form, or gut cells, or immune cells. “Anything you imagine cells can do may occur when a cell gets a Notch signal,” says Jon Aster, a physician-scientist at Brigham and Women’s Hospital in Boston, who works on the Notch-cancer connection. “It’s an alluring pathway — and a daunting and sometimes confusing one, too.”
The precise amounts of Notch activity are crucial. Too much or too little and the function of cells, and the body as a whole, can go awry. Several human disorders are linked to mutations in genes that encode Notch (we have four such genes, and four distinct Notch proteins). Babies born with Adams-Oliver syndrome have malformed limbs and skin; those with Alagille syndrome have defects of the liver, heart and other organs.
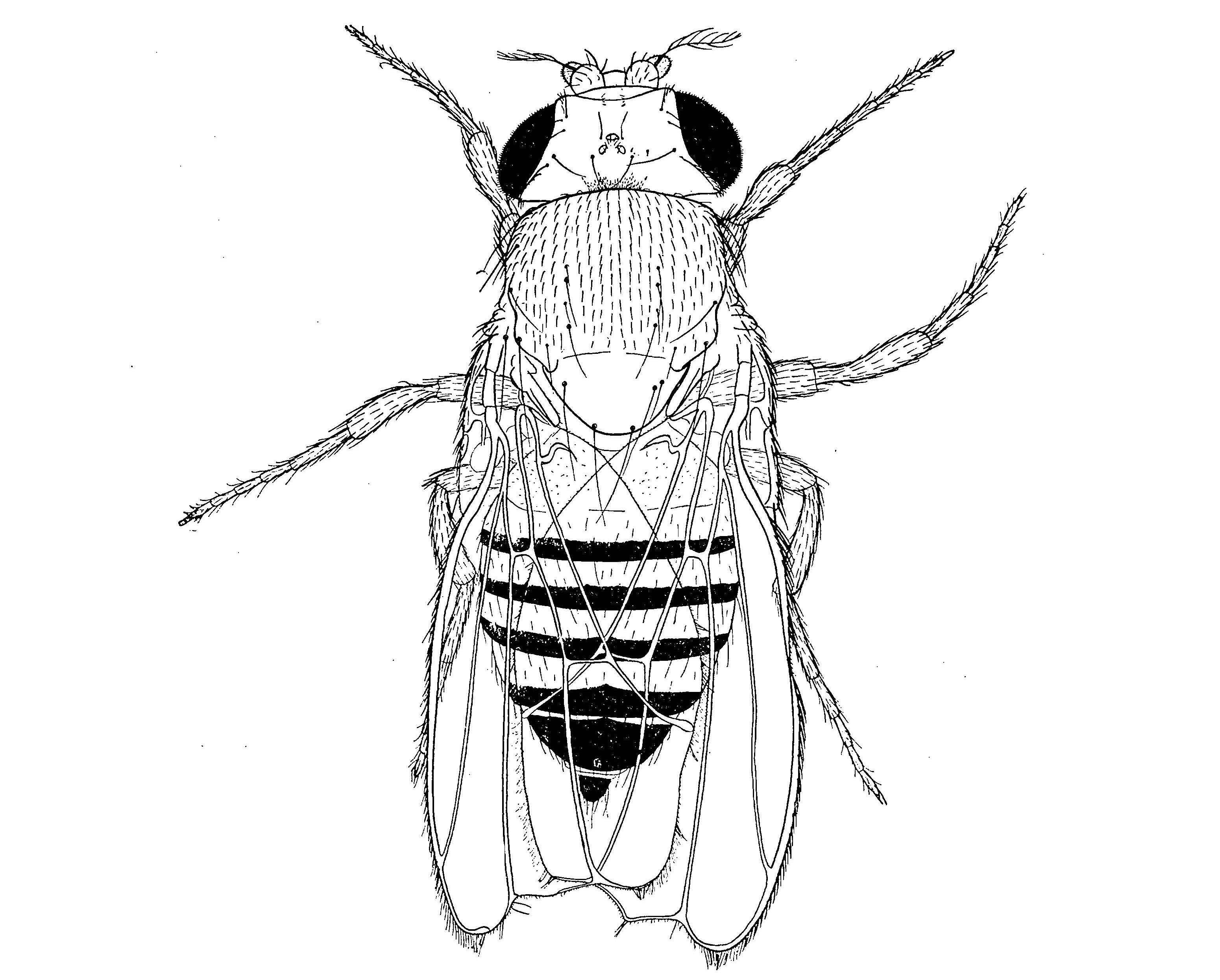
A century ago, pioneering geneticist Thomas Hunt Morgan studied flies with odd ‘notches’ on the tips of their wings. Today, we know that Notch genes play myriad roles in animal bodies.
CREDIT: T.H. MORGAN/AMERICAN NATURALIST
Scientists also now know that defects in the Notch pathway are involved in many human cancers. When Notch is active when it shouldn’t be, the result can be childhood leukemia, other blood cancers and certain types of breast and salivary gland cancer. Conversely, when Notch isn’t active when it should be, certain skin, head and neck and brain cancers can result.
Defects in the Notch pathway contribute in many distinct ways: They can turn on cancer-causing genes, such as one called Myc that drives cell proliferation. They may put cancer cells into hibernation and thus help them survive chemotherapy. Or the defects may stop tumor cells from committing suicide or encourage the formation of cancer stem cells that sustain tumor growth.
Based on this coalescing knowledge, drug companies and academic labs are tinkering with various therapies that could block inappropriate Notch activity or bring it back when it’s needed. They’re using an array of different molecules to amplify or block the signal: Some are antibodies that bind to a Notch receptor or its activator; others stop Notch from being clipped into its active form. Still others stop that active, clipped Notch from turning on genes in the nucleus. Scores of small trials are underway but it’s still early, and the results have been scattershot: Some patients in trials have responded very well, but others have not. In one recent trial, patients actually did worse.
“This is not chemotherapy, it doesn’t just massacre cells no matter what their genetics is. It needs to be used in situations where it is likely to work.”
Lucio MieleThere are no shortcuts to be had here, says physician-scientist Lucio Miele of Louisiana State University in New Orleans, who has conducted Notch clinical trials. Instead, the basic biology must be dutifully teased out, ways devised to cut down on side effects and — perhaps above all — patients selected with care and matched well to treatments. “This is not chemotherapy, it doesn’t just massacre cells no matter what their genetics is,” Miele says. “It needs to be used in situations where it is likely to work.”
Notch therapies hold promise for more than just cancer. Scientists are also eyeing them as ways to treat immune problems, such as graft-versus-host disease, a feared side effect of transplantation wherein immune T cells in grafted tissues such as bone marrow attack a recipient patient’s body. The disease is a scourge of the bone marrow transplant world, says hematologist and researcher Ivan Maillard of the University of Michigan in Ann Arbor. Sometimes it’s chronic — slowly stiffening joints, causing skin to ulcerate, attacking the liver and lungs. Sometimes it’s acute, rapidly attacking body tissues — and if patients don’t respond well to immune-suppressive drugs to tamp down that roiling inflammation, they generally die. “There’s a huge unmet need,” Maillard says of graft-versus-host disease. “You never know who is going to get it. It’s a roll of the dice. You hope for the best.”
It’s been difficult, he adds, to find treatments that will block graft-versus-host disease and still retain the good things a bone marrow transplant provides a cancer patient — chiefly, that T cells in the graft will kill remaining tumor cells. Notch might offer this chance. Maillard’s group has shown, in mice, that blocking one of the four Notch receptors tamps down graft-versus-host disease after a bone marrow transplant, while keeping the benefits intact. Surprisingly, the blockade needs to be up only for a short time: about 48 hours.
That’s encouraging, Maillard says. It suggests that, if the system works similarly in people, doctors could give a patient a transplant, infuse a Notch-blocker for a pulse of time, then permit Notch activity to come back online. It would make side effects in other tissues far less likely.
“It’s incomprehensible to me that there will not be a role for Notch therapeutics in the treatment of human disease.”
Jon AsterIndeed, side effects are a challenge for those who would design Notch therapies. This is only to be expected for a set of proteins that do so many jobs in the body. But the four human Notch receptors are not identical, and researchers think that they can render therapies far more specific and safe through further learning and tinkering. (Some tinkerers, meanwhile, want to take Notch in entirely new directions by engineering synthetic Notch proteins that could perform a broad range of novel functions.) “It’s incomprehensible to me that there will not be a role for Notch therapeutics in the treatment of human disease,” says Aster. “This is just such a broadly important pathway.”