We’ve known for a long time that viruses aren’t all bad. Many just hitchhike through living organisms without causing any problems. And some are beneficial, even necessary, for life on Earth. We wouldn’t be here without them, says Marilyn Roossinck of Pennsylvania State University.
Roossinck loves viruses so much she wrote the book “Virus: An Illustrated Guide to 101 Incredible Microbes” (Princeton University Press, 2016). She also coauthored the article “Symbiosis: Viruses as Intimate Partners,” in the 2017 Annual Review of Virology.
And the association is intimate. Viruses can only survive by entering and copying themselves inside a living cell. They use the machinery of the host cell to replicate their genetic material and produce more viral particles.
More than 200 kinds of viruses are known to infect people, and scientists estimate that a few hundred thousand infect other mammals. Add in those that infect plants, bacteria and other organisms, and viruses are big players in the biosphere. Overall, scientists propose there are 1031 individual viruses on the planet — that’s 10 nonillion of them.
Knowable Magazine caught up with Roossinck to ask about how viruses benefit plants and people, and to discuss the plant viruses she’s most excited about right now.
Why do you, personally, study viruses?
I fell in love with viruses when I was an undergraduate student, taking a microbiology course. A virus of bacteria called lambda was the first virus I met, and it looked so cool, like a little spaceship. I was amazed at the intricacy of their relationships with their hosts and I made a decision, at that moment, that I was going to be a virologist. I still love them. They’re just amazing.
What do we know today about viral numbers and diversity on Earth?
Viruses are the most abundant and diverse beings on the planet, and they’re found everywhere. We probably still know only a small fraction of the viruses that exist, but we’re learning more every day. We keep accumulating more and more virus-like genetic sequences from what are called metagenomics studies — where scientists are just sampling everything in an environment.
What we are really lacking is an understanding of what any of these things we’ve sequenced do, or how they might be related to each other. Often, we don’t even know what their host is.
Do we have any idea how many viruses a single person hosts at a given time?
That’s a tough question to answer these days. Scientists are finding lots and lots of viruses in metagenomics studies of the human gut, and a lot of these viruses are probably infecting the bacteria in the gut, rather than the person’s cells. I wouldn’t like to put a number on it, but when people ask I usually say, “a bazillion.” We don’t know, there’s a lot.
And do we know how many viruses are bad, good or neutral for their host?
I like to say 1 percent are pathogens, which harm their host, but I think that’s probably a very high estimate. That’s based on early studies with simian [ape and monkey] viruses, which were studied a lot during early days in molecular virology. There were 80-something of these simian viruses they discovered and they were numbered SV1, SV2, etc. Of those, SV40 is the only one that’s had very much study done, and that’s because it turned out to be a pathogen, causing tumors in mice. None of the rest of them had any effect on the host they were tested in. That’s where I get my “about 1 percent.”
You were originally inspired by bacterial viruses, but in your research today, do you have a favorite virus, or one that particularly interests you?
I would say, more like a favorite class of viruses. For the last few years I’ve become really fascinated with what are called “persistent plant viruses.” This is largely because when I was doing virus discovery work, we found they were the most common type of viruses in wild plants. They’re also very common in crops, but nobody had studied them very much.
These are viruses that have infected their hosts for, probably, thousands of years. They’re passed, through the seed, into the next generation, and then they infect every single cell in the host. They don’t pass between adult plants, so far as we know.
They’re usually found in pretty low numbers, and we don’t know very much about what they do. In some cases, we know they benefit the plant. For example, white clover cryptic virus affects nodulation in legumes. Legumes normally form nodules of bacteria, in their roots, to help them take up nitrogen from the atmosphere. But doing so is costly for the plant. In virus-infected legumes, when there’s enough nitrogen in the soil, then they don’t form nodules, and that’s a benefit to the plant.
We’ve been studying another one called pepper cryptic virus. It’s a very hard thing to prove, but it seems like virus-infected seeds have a lot longer longevity than uninfected ones. After a couple of years, the uninfected seeds don’t germinate, whereas the infected cells last for many years.
Because these kinds of viruses are in a lot of crop plants, we think they probably confer some benefit, like perhaps that longevity, that made farmers breed and plant them during early agriculture.
What are some other ways viruses benefit their hosts?
Many plant viruses confer drought tolerance or cold tolerance to plants. We don’t always know how this works but, for example, elevated sugar is very common in virus-infected plants. More sugar would allow the plant cells to retain more water, protecting them from drought. And you know, things that are really sweet freeze slowly, so extra sugar would make plants cold-resistant.
And in animals, actually in mice, herpes viruses confer resistance against bubonic plague. That’s because the herpes virus, dormant in the mouse, turns up the mouse’s immune system and makes it better able to fight the plague.
Similarly, in people, hepatitis G virus may offer some protection against AIDS. Hepatitis G, now called pegivirus or GB virus C, is quite common in humans, and isn’t known to cause any disease. But it does affect the immune system in a variety of ways. If people are infected with hepatitis G first, and then HIV, it takes longer for it the HIV to progress to AIDS.
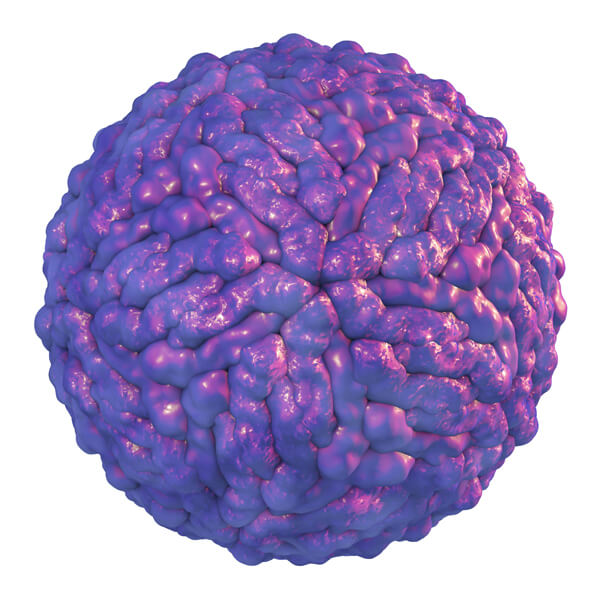
Pegivirus, illustrated here, seems to bring benefits, at least in people later infected with HIV. Formerly known as hepatitis G, pegivirus affects the immune system in a way that has been shown to delay the onset of full-blown AIDS.
CREDIT: KATERYNA KON / SHUTTERSTOCK
Some viruses even become an integral part of their host, with their genes incorporated into the host’s DNA. Can you give some examples of that?
We call that symbiogenesis. “Symbiogenesis” has been used to refer to things like the mitochondria or chloroplasts, that were of bacterial origin and then were involved in the evolution of eukaryotic life. But the more genomes we sequence, the more we find viruses. And not just retroviruses, which we know integrate into host genomes during their normal life cycle, but all kinds of RNA viruses, small DNA viruses. There are lots of things that viruses could do in a host genome. For example, they might turn genes on or off.
I think the very first example of symbiogenesis that was studied by scientists has to do with the breakdown of starches by our saliva. Most of us did this little experiment in elementary school: You chew bread until it turns sweet. That’s because an enzyme called amylase, in our saliva, breaks the starch down into sugars. A more obvious place for amylase is in the gut, to break down food. The reason it’s made in the salivary glands too is because viral genetic material integrated in front of the amylase gene, and turns it on in salivary glands.
The most dramatic example of symbiogenesis is in the evolution of the mammalian placenta. A protein called syncytin fuses cells together to make a placenta, and it evolved from a virus protein. So the gene for this protein, normally part of the membrane that surrounds a virus, integrated into the mammalian genome during the evolution of the placenta.
What else is in the future for virology?
I would say that in the future, we’re going to find a lot of things that we can use viruses for, as beneficial agents. For example, I know there is some work going on right now to use viruses to get rid of bacteria that are infecting crop plants.
But the biggest obstacle, I would say, is that people are still a little afraid of viruses. If you say to somebody, “Why don’t you ingest some hepatitis G virus because it would protect you from AIDS?” — you know, probably nobody’s going to do it. Even among some virologists, that bias against viruses is still a hurdle.
You have written that viruses are central to life. Can you imagine what would a virus-free world look like?
There wouldn’t be a world. I think that viruses are probably remnants of the original life form. It’s high time they got the credit they’re due.