There’s a reason the world has more hobbyist bird-watchers than, say, rodent-watchers: Birds are unusually fascinating creatures. Biologists aren’t immune to their charms — they’ve long been working to understand why and how the creatures come by their flashy colors, varied shapes, florid songs and intricate behaviors. Those aren’t easy questions to answer, given the thousands of genes — many of unknown function — in a typical bird genome. Searching for the gene or genes behind a given trait can feel like trying to map out a vast landscape with a dim flashlight.
But now geneticists have turned on the floodlights thanks to new technologies that make sequencing entire genomes fast and cheap. Able to study the whole genome at once, scientists have unprecedented power to understand the biology of birds, from their evolutionary origins to their many eye-catching adaptations — and even to follow one of evolution’s most important events, the origin of new species.
“Having a genome is like opening up a gate,” says Gustavo Bravo, an evolutionary biologist at Alexander von Humboldt Institute in Boyacá, Colombia, who coauthored a look at what we’ve learned from bird genomes in the 2021 Annual Review of Ecology, Evolution, and Systematics.
The torrent of new genomes is truly astonishing. Just over a decade ago, in 2010, scientists had sequenced just two bird genomes, of the chicken and the zebra finch. Five years ago, there were almost 100 completed genomes — and as of early February 2022, genomes had been completed for 542 species of birds, including at least one from every order.
Almost as soon as ornithologists got hold of this mountain of data, they started using it to sort out the messy evolutionary tree of birds. The fossil record tells us that when the rest of the dinosaurs went extinct about 66 million years ago, the little dinosaur group we now call birds evolved quickly to fill the empty ecological niches, with most major groups of birds appearing within a few million years.
This burst of evolution happened so fast, and so long ago, that instead of an orderly branching pattern, the tree looks like a multi-stemmed shrub, leaving ornithologists struggling to identify which branches are most closely related. (Two ancient groups, the ostrich-like birds and the fowl, such as modern ducks and chickens, had branched off earlier, so their relationship to the others is less confused.)
Whole genomes may help crack this “wall of death,” as researchers wryly call it, because the genomes contain so many types of information — not just the genes themselves, but DNA regions that regulate the genes, spacers between genes or within them, the hulks of old viruses that inserted themselves into genomes long ago, and much more. Each species’ genome also reflects rare events like gene duplications or deletions, the breaking or fusing of chromosomes, and places where part of a chromosome gets flipped back to front. “A genome is a mash-up of many different histories resulting from many processes,” Bravo says — ones that can sometimes carry crucial information about evolutionary history.
As genomes rapidly become quicker and cheaper to sequence, the number of species sequenced has grown dramatically over the past few years, especially for birds, fish and mammals.
Researchers have not yet broken through the wall of death completely. But already, some surprises are emerging. For example, earlier evolutionary trees based on genes that carry instructions for making proteins suggest that waterbirds such as pelicans, herons and flamingoes form a single cluster, while trees based on other elements of the genome show these birds widely scattered among other bird groups. If the latter pattern proves correct, then the similarities among waterbirds’ protein-coding genes could be a red herring. “Living in water needs specific adaptations,” Bravo explains, so waterbirds’ genes could have arrived independently at similar sequences.
Whatever the outcome of this specific case, it’s clear that the tree you build can depend on which parts of the genome — which “markers,” in the argot of geneticists — you use. The best way out of this problem may be to look at as many types of genome data as possible. “There is no perfect marker,” says Alexander Suh, an evolutionary biologist at the University of East Anglia in the UK. Instead, researchers need to look at as many different types of markers as they can, to follow the weight of the evidence. And that’s only possible with complete or nearly complete genomes.
Tracking adaptations
Bird genomes are good for more than just de-jumbling evolutionary trees, however. Sometimes, scientists are interested in specific adaptations: What genes help a bird to colonize a new habitat? What accounts for the differences in colors and patterns in the plumage of closely related birds? Here, too, looking at entire genomes provides a much more powerful approach. “We don’t need any preconceived ideas of what to look for,” says Sangeet Lamichhaney, an evolutionary biologist at Kent State University in Ohio. “We can just compare the genomes and ask, what are the differences?”
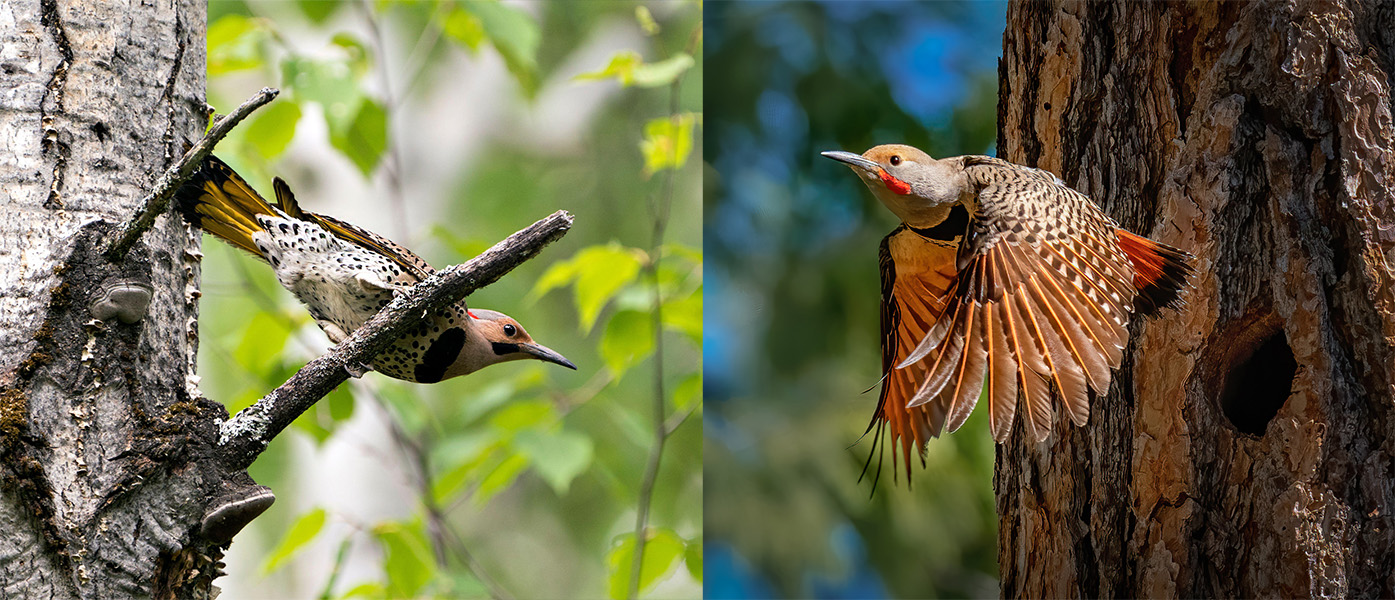
These two Northern flickers, which differ in the color of their wing and tail feather shafts, were once thought to be separate species. Comparing the genomes of the two birds is helping biologists to understand what lies behind their color differences.
CREDIT: NORTHERN FLICKER YELLOW-SHAFTED / ALAMY; ALICE CAHILL / FLICKR
Consider, for example, the antbirds, a group of small insect-eating songbirds native to South and Central America. Most of the roughly 240 species live in humid lowland forests, but a handful of species have — independently — colonized drier habitats. Bravo and his colleagues chose a few of these species and compared their genomes to closely related species from wetter forests, hoping to find places in the DNA where the dry-habitat species differ consistently from their jungle cousins. If several species independently show changes in the same part of the genome, they figured, an especially important gene must live there.
Sure enough, they found some differences: ones linked to genes that are important in water balance and temperature regulation in adult birds and, surprisingly, even in the egg. But most differences were not in the genes themselves — that is, the parts that actually code for the proteins that produce the traits. Instead, the differences turned up in regulatory regions that control gene activity, turning production of a given protein up or down, or perhaps making the gene active in different tissues. In other words, antbirds use the same kit of tools in dry habitats as in moist ones, but they deploy the tools differently. (Bravo’s work has not yet been published.)
“Using whole genomes and comparative methods allows us a different way into finding associations between traits and genes,” Bravo says. “If I only had sequences of a few [genes], I would never be able to find these things.” In the future, he says, researchers could use gene-editing tools to modify the regulatory regions and see if this alters the arid adaptations.
Other researchers are ransacking genomes for genes related to coloration. One group, for example, is trying to spot the genes that make the difference between the yellow throats of Audubon’s warblers and the white throats of closely related Myrtle warblers. Another team is attempting to understand the genes behind the coloration of two subspecies of flickers (types of North American woodpeckers), one with red feather shafts and the other with yellow ones. In each case, the researchers are exploiting the tendency of the two birds to hybridize, leading to novel mixes of genes that can help to identify which genes determine the color pattern.
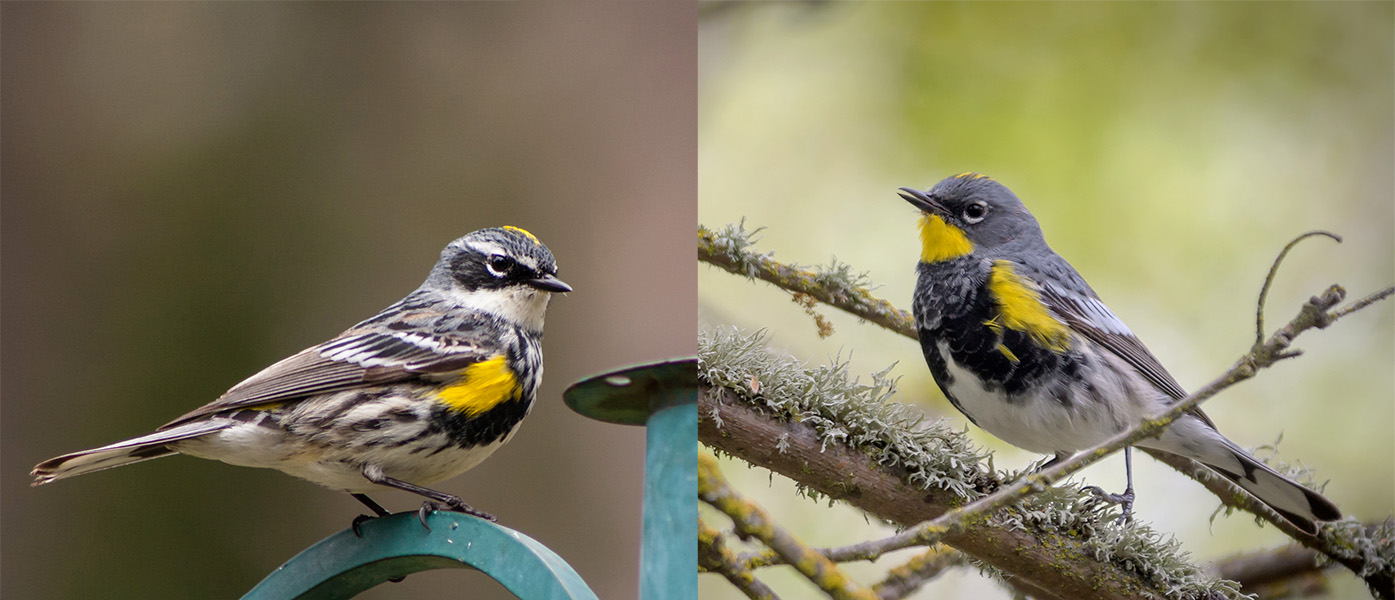
The Myrtle warbler (left) and Audubon’s warbler (right), two subspecies of the Yellow-rumped warbler, differ in coloration, especially in their throat patches. Researchers are exploring the birds’ genomes to identify the genes responsible for these color differences.
CREDIT: PAUL VANDERWERF / FLICKR; BECKY MATSUBARA / FLICKR
Origin of species
Genomes are even shining a fresh light on one of the most important, but most difficult to study, events in evolution: the origin of new species. And, appropriately, it’s happening with Darwin’s finches in the Galapagos Islands. “These birds started the idea in Darwin of evolution,” says Lamichhaney. “They are the textbook example of speciation and adaptation.”
In 1981, evolutionary biologists Peter and Rosemary Grant — the world’s experts on Darwin’s finches, having studied them in the Galapagos for decades — noticed a new finch on the tiny island of Daphne Major. Since Darwin’s finches are not skilled long-distance fliers, they assumed it had come from the nearby island of Santa Cruz. The immigrant and its offspring interbred with the locals for a generation or two, but then the hybrids began mating exclusively among themselves — becoming, essentially, a new species.
After the first Darwin’s finch genome was sequenced in 2012 (Geospiza fortis, the original resident species on Daphne Major), the Grants approached Lamichhaney and his colleagues, asking them to apply their genomics skills to the Grants’ stored genetic samples from the birds. Sequencing quickly showed that the immigrant finch hadn’t come from nearby Santa Cruz after all, but from far-off Española. “We thought we were doing something wrong,” Lamichhaney recalls. “We thought there was no chance the bird came from that distant island.” But it had, as its genome testified.
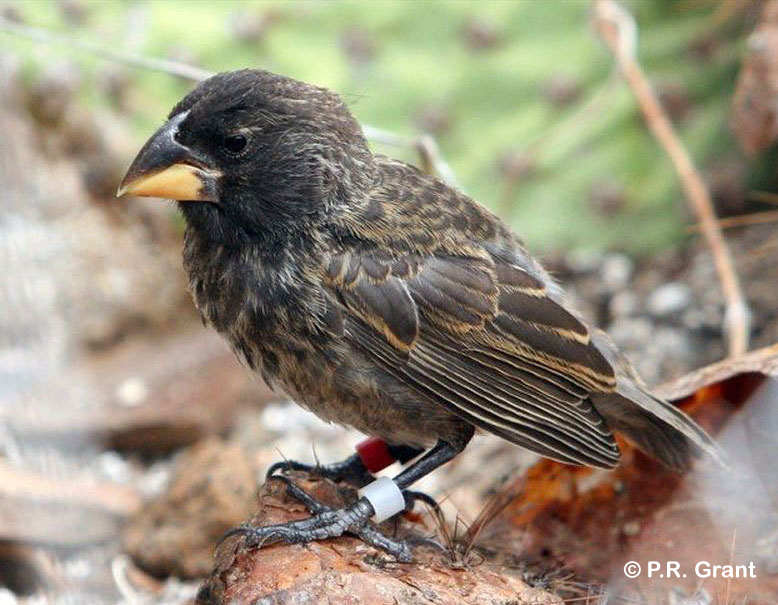
In 1981, a new Darwin’s finch showed up on a small island in the Galapagos islands and interbred with the resident finches. Soon its descendants, including the one shown here, formed a distinct lineage that bred exclusively among themselves — a new species. Thanks to genomics, researchers now know where that original migrant came from and are beginning to work out what makes the new lineage distinct.
CREDIT: PETER GRANT
The next step was to trace how the hybrids’ genomes had changed as they became a distinct species. This is still a work in progress, Lamichhaney notes, but already it’s clear from genomic comparisons that two genes — ALX1, which affects beak shape, and HMGA2, which affects beak size — played a crucial role in making the hybrids different enough to not interbreed with the local finches. (Beaks play an important role in the mating behavior of Darwin’s finches.) The researchers expect, however, that other genetic changes related to song type might also be important. “This is the great power of genomics,” Lamichhaney says. “With the entire genome, you can look at the network of regulation.”
Scientists can do many other things with genomes, too, from identifying populations in need of conservation to exploring how the structure of genomes themselves changes through evolution. But with genome sequences already in hand for at least one species from every order and most families of birds, ornithologists are nearing the end of the first big genome boom.
At this point, they probably have enough genomes in hand to answer most of the big questions of bird evolution — but every new one will still be valuable for working out the fine details of evolution within families and species, Bravo says.
And, he adds, “there’s room for surprises.”