The ornately folded outer layer of the human brain, the cerebral cortex, has long received nearly all the credit for our ability to perform complex cognitive tasks such as composing a sonata, imagining the plot of a novel or reflecting on our own thoughts. One explanation for how we got these abilities is that the cortex rapidly expanded relative to body size as primates evolved — the human cortex has 10 times the surface area of a monkey’s cortex, for example, and 1,000 times that of a mouse.
But the cortex is not the only brain region that has gotten bigger and more complex throughout evolution. Nestled beneath the cortex, a pair of egg-shaped structures called the thalamus has also grown, and its wiring became much more intricate as mammals diverged from reptiles. The thalamus — from the Greek thalamos, or inner chamber — transmits 98 percent of sensory information to the cortex, including vision, taste, touch and balance; the only sense that doesn’t pass through this brain region is smell. The thalamus also conducts motor signals and relays information from the brain stem to the cortex, coordinating shifts in consciousness such as waking up and falling asleep.
Scientists have known for decades that the thalamus faithfully transmits information about the visual world from the retina to the cortex, leading to the impression that it is largely a messenger of sensory information rather than a center of complex cognition itself. But that limited, passive view of the thalamus is outdated, maintains Michael Halassa, a neuroscientist at the Massachusetts Institute of Technology who recently coauthored (with Ralf D. Wimmer and Rajeev V. Rikhye) an article in the Annual Review of Neuroscience exploring the thalamus’s role.
In rodents, humans and other mammals, Halassa says, the thalamus is much more than just a passive gatekeeper for sensory input. Instead, it plays an active role in orchestrating cortical activity, telling the cortex what tasks it should carry out in different contexts. For example, it directs the split-second recalibration required when an American in England must decide whether to look right or left before crossing the street where cars drive on the “wrong” side of the road. This ability to quickly adapt behavior to new situations and context likely arose from changes in neuronal wiring between the thalamus and the cortex. Those changes also may help explain why humans are able to act on internal representations of the outside world, such as memory and imagination, Halassa says.
Knowable Magazine asked Halassa to discuss how work in his and other labs is changing the traditional view of the thalamus. This conversation has been edited for length and clarity.
How did you become interested in studying the brain, and the thalamus in particular?Watching The Matrix. I thought that film was one of the most unbelievable demonstrations of what it means to have a mind – especially a mind that is plugged into virtual reality. What does it mean that there are possibly infinite numbers of ways in which the outside world can be viewed and interpreted? How can the brain generate a mind? These are the sorts questions that drove me to study neuroscience.
As a graduate student I was interested in studying consciousness, and I ended up studying the basic mechanisms of sleep. There are special territories within the thalamus that are highly active and interesting during sleep, and help to orchestrate major shifts in brain state, such as dozing off or waking up. In my post-doc, I focused on the interactions between the thalamus and cortex, which is the current topic of my research.
What is the thalamus, and how is it connected to the cortex?The thalamus is made up of round, egg-like structures under the cortex, one on each side. In mammals it is connected to all cortical areas, and there are two-way tracts of neurons that go from the cortex to the thalamus, and from the thalamus to the cortex. If you go to the back of the brain, where the cortex processes visual information, you see connections to the back of the thalamus, which transmits visual signals. The front of the cortex, where higher level cognition occurs, is also connected to the more anterior, or frontal, part of the thalamus.
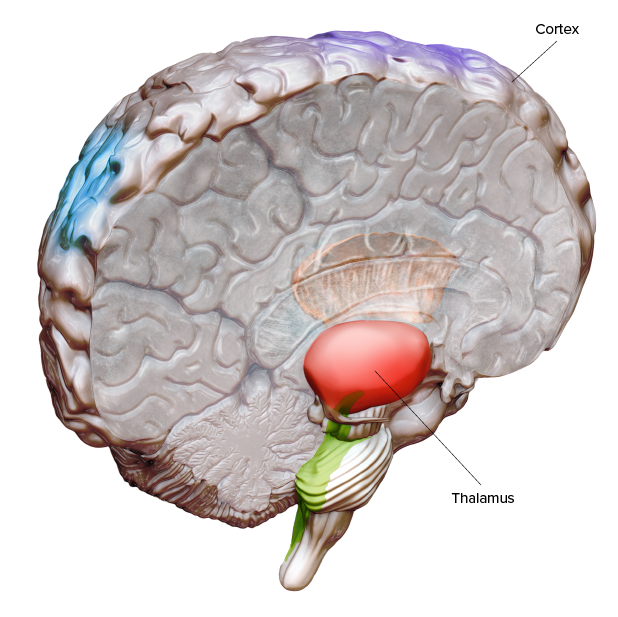
The thalamus (red) has long been considered primarily a relay station for sensory information, but growing research suggests that it also increases the brain’s ability to adapt to new contexts, enabling the use of memory and imagination when deciding how to act.
CREDIT: NICOLLE R. FULLER / SAYOSTUDIO
In reptiles and amphibians and fish there is really not much of a cortex. Instead, there’s a structure called the pallium, which is a cortical analog, but it doesn’t seem to send a lot of projections to the thalamus. In these species, it seems like the thalamus is mostly telling the pallium about the state of the outside world. But as you go through evolution, the thalamus starts getting a lot of inputs from the cortex. It’s as if cortical neurons began to “invade” back down into the thalamus, creating a two-way flow of information. You could think of the cortex as a series of memory banks, and the thalamus as a pointer which determines what tasks should be deployed in different contexts. But there’s something potentially romantic about this role for the thalamus — you’re starting to free up the organism from the immediacy of the outside world, allowing it to base its actions on internal representations, including memory.
The traditional view of the thalamus’s role is that it is primarily a relay station for sensory information, such as vision. How did that view come about?Just like there are many cortical areas, the thalamic areas are segregated into different parts and each interacts with a different part of the cortex. The sensory areas are best understood because they are easier to study, and the best studied part of the thalamus is the lateral geniculate nucleus [LGN], which gets inputs from the retina in the eye and projects to the visual cortex at the back of the brain.
In primates, the LGN functions like a relay, meaning that it takes inputs from the retina, but doesn’t do a ton with them. The retina is like a camera, which can pixelize the outside world. Light hits the camera, or retina, and the light intensities from different points in the visual field are encoded to pixel intensities. The interesting transformations of visual information — where you go from pixel intensities to actually seeing shapes and objects — don’t start until the primary visual cortex.
What’s so interesting about primate vision and visual cognition is that when we interact with the world, we’re not just interacting with pixel intensities, we’re building internal representations of objects. That’s why you can categorize any cup you see, even if you've never actually seen that particular cup before, and it looks different from other cups. Neurons that can specifically recognize male or female faces, or respond only to a photo of Jennifer Aniston — all that exciting stuff happens in the cortex, which is part of the reason that the thalamus has been sort of shoved to the side.
But there are many other areas of the thalamus besides the LGN and other sensory areas that interact with the cortex, including areas involved in motor control and cognition. Now we are studying those too.
What evidence suggests that the thalamus is doing something more interesting than previously thought?In mice, we have found that neurons in other parts of the thalamus do not operate like neurons in the LGN. This is particularly true for the more frontal parts of the thalamus like the mediodorsal thalamus, which is connected to the prefrontal cortex.
The prefrontal cortex is thought to be one of the most complicated cognitive areas of the cortex — it performs tasks that are even more complicated than face recognition. In humans, for example, it enables our ability to draw parallels, to imagine things that we’ve never seen, and to innovate. Based on our animal studies, we think that the mediodorsal thalamus is helping the brain narrow down its search for the correct behavior or response, so that it can quickly adapt as the environment or situation changes. It takes incomplete data from the cortex, then uses that information to determine which tasks the cortex should perform.
Neurons in the lateral geniculate nucleus (LGN) of the thalamus have fairly simple structures and a straightforward task: transmitting visual data from the retina to the cortex. Neurons in the mediodorsal (MD) region of the thalamus, in contrast, have much more complicated branches, and appear to perform more sophisticated neural computations. (The colors in the MD neruons reveal the neurons link to different layers of the cortex, rather than terminating mainly in the upper layers of visual cortex.)
Being able to switch responses based on context can mean a lot of different things. When you see a flash of red light at the traffic intersection, for example, you hit the brakes. That’s almost reflexive. But you can behave differently when you see a flash of red light on a dance floor, or even in a boxing ring, where a flashing red light is used to start each round. The shift in the brain — the shift in what parts of the brain become engaged to enable the appropriate behavior — is what I think the thalamus can afford. It’s about being able to get another cortical area online fast enough to allow for this context-dependent processing.
We don’t have any data on this in humans. But I have colleagues at MIT who are now studying this kind of context-processing in primates, recording in the motor cortex and the thalamus of the monkey. They can also see evidence for thalamic control of motor-cortical states, very similar to what we’ve been describing in the mouse.
What happens when the thalamus is damaged in people?Lesioning the thalamus in a person can cause loss of consciousness or selective perceptual abnormalities or really bad memory problems. If you lesion the mediodorsal thalamus, you have executive deficits or memory problems. In schizophrenia, the structural connections between the prefrontal cortex and the mediodorsal thalamus — these two-way streets — are also changed. I’m really interested in what insights we can get from animals that would be able to tell us what’s changing in the thalamus and how the schizophrenic brain builds an internal model of the world.
What are other potential applications of your research?AI is one potential application. I think that if we want to create artificial intelligence it will be very important for those architectures to be able to generate neural dynamics the way our brains do. There are really important cognitive functions that are attributed to the thalamus, so if we understand those architectures it has the potential to change the way we design artificial agents, by building computers with connections that mimic the real neural pathways between the thalamus and cortex.
I’m also interested in the interface between brain science and the humanities. On a fundamental level, art is context. For example, if you look at a face with distorted features in a Picasso painting, you register that as sort of OK, because you know the context is art, and art is a context in which familiar norms do not have to be respected. If you see the same distorted face in real life, however, you are likely to respond differently. We don’t know exactly if this is what the thalamus is doing in humans, so this is an inference. But we believe the instructions for what tasks the brain should perform in situations like that are in the thalamus.