Plant biologist Pamela Ronald is concerned with the pressing problem of feeding the world without destroying it. The question of how to grow enough food for an expanding global population has grown more urgent in the face of climate change. And it’s only made harder, she says, by the push-back against the use of the genetic tools now at scientists’ disposal.
Ronald’s views have emerged from nearly 30 years of research on how plants resist disease and tolerate stress, work that is ongoing in her lab at the University of California, Davis. Much of that work has focused on rice, a staple crop that feeds nearly half the globe. While she’s an outspoken advocate for using genetic engineering to modify crops — her TED Talk The Case for Engineering Our Food has been translated into 26 languages and watched more than 1.7 million times — she’s also married to an organic farmer, Raoul Adamchak. Together, they wrote the book Tomorrow’s Table: Organic Farming, Genetics, and the Future of Food, exploring how the best of both approaches might be needed for long-term sustainability.
We spoke with Ronald about her research and her views on genetic modification and its place in the sustainable agriculture toolbox. This conversation has been edited for length and clarity.
How do genetically modified crops fit into the sustainable agriculture landscape?
Sustainable agriculture has three pillars: social, economic and environmental. It creates food that’s nutritious, it allows farmers to reduce the amount of land and water they use, to foster soil fertility and genetic diversity, and to reduce toxic inputs. And it enhances food security for the very poorest farmers and families in the world. So, for example, if you can breed resistance into a plant, whether through conventional or genetic engineering, and that means you can reduce the amount of sprayed chemicals you use, that’s part of sustainable agriculture.
Any type of agriculture is pretty challenging. Most farmers are trying to move their farm toward more sustainable approaches. Unfortunately, there’s no magic bullet because farmers in different regions of the world face different challenges, grow different crops and have different markets.
The book you and your husband cowrote is titled Tomorrow’s Table. What does tomorrow’s table look like to you?
In the book, we describe what’s on our table and explain how the foods were developed — the kinds of genetic techniques and organic farming techniques used to produce that food. We try to give the reader an idea of what geneticists do and what organic farmers do. We have a number of recipes.
But the book isn’t about nutrition, it’s about: How do we produce and provide that nutritious food with minimal environmental impacts? How do we ensure that farmers and rural communities can afford the food? How do we address this critical challenge of our time: to produce sufficient, nourishing food without further devastating the environment? There are a lot of issues, a lot of people on the globe right now, and even more in the future. They all need to eat.
Sticky “mutant” rice, included in this recipe from the book Pamela Ronald and her husband wrote, came into being more than a thousand years ago. The stickiness arose thanks to a spontaneous genetic mutation that disrupted the gene for making the starch amylose, which helps make non-sticky rice fluffy. The recipe juxtaposes that ancient genetic modification with a more modern one: genetically engineered papaya, which farmers began planting in the late 1990s after papaya ringspot virus decimated orchards.
Does your husband have a different view of the future of food?
It’s a shared view. We both think people should focus on the challenges and not get distracted by the concept of genes in our food. We really want to use all the tools that are available and use scientific-based farming practices, such as those that minimize pests and disease. There are many organic farming practices that are very useful, such as crop rotation.
It’s the combination of farming strategies and genetic strategies that are going to continue to be quite important for producing our food and moving forward to a sustainable farming future. Farming is destructive. But, as my husband says, we farm because we have to eat. Some people say, well, let’s change our diets, or reduce waste. Those are both important, but we still need technological change. All these aspects are even more critical as the population continues to grow.
A lot of your research has focused on rice, a hugely important staple crop. Did you always want to work on rice?
I was working on peppers and tomatoes as a graduate student at UC Berkeley and as I was making the transition to a postdoc, I thought, what do I want to do, because this may last my whole career. And I decided to work on rice because it feeds half the world’s people. It’s also a very good genetic system; it’s easy to do genetic analysis of rice. So I thought if we can make any kind of incremental advance we could potentially help millions of people.
One of those advances has been the development of flood-resistant rice. I’ve seen so many photos of rice paddies flooded with water, doesn’t rice tolerate flooding?
The rice plants that many of us are familiar with grow well in standing water. But most rice plants will die if they are completely submerged for more than three days. When the leaves are submerged, they can’t carry out photosynthesis. My UC Davis colleague David Mackill was working with this ancient variety of rice, discovered at the International Rice Research Institute, that could be completely submerged in water for two weeks, and then can start to grow again when the water is removed. So this was very, very exciting.
Breeders then tried to use conventional breeding to introduce this trait from the ancient variety into varieties grown by farmers. But when you cross-pollinate with another variety, even though it has a nice trait, you can bring a lot of other traits you don’t want. So, the result from conventional breeding were rice varieties that were rejected by farmers because they had traits that the farmers did not want such as reduced yield, or a change in the texture of the rice grain.
How did you tackle the problem?
First, we carried out the initial work of isolating the flood-tolerance gene, called Sub1a, from the ancient variety. Then we introduced the gene into a model rice plant using genetic engineering. We then grew up those plants and submerged them, in large tanks in our greenhouses for two weeks.
The plants that carried the Sub1a gene were very robust; you could see the difference right away. Plants without Sub1a turned yellow, had very long leaves and soon died. This is because when the leaves try to grow out of the water, they deplete their chlorophyll content and energy reserves. But the plants that carry the Sub1a gene just stay kind of metabolically inert — they don’t grow very fast, they just kind of wait out the flood. And when the flood’s gone, they start to regrow. The Sub1 plants remained green and healthy, indicating we had indeed isolated the correct gene.
Is Sub1 rice now being grown by farmers?
Yes. As I described we used genetic engineering tools to isolate and validate the submergence-tolerance gene in the greenhouse. That genetic knowledge was then used to develop a flood-tolerant variety through a different approach called marker-assisted breeding. That work was done by the International Rice Research Institute. The ancient, flood-tolerant variety was cross-pollinated with a modern variety that farmers like because of its flavor and high yields. Seeds derived from those hybrids were planted, and tested for the preferred genetic fingerprint that included Sub1a but did not carry genes from the ancient variety that affected traits important to the farmers.
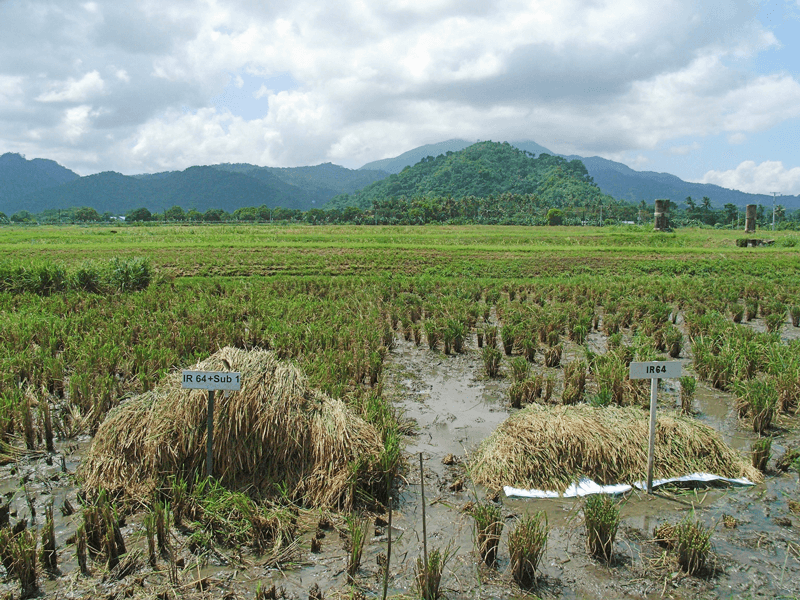
Rice bred to contain the Sub1a gene can survive even when completely submerged for 17 days. This flood-tolerant rice yielded 3.8 tons per hectare (pile on left), compared with 1.4 tons per hectare for the same variety lacking the flood-tolerant gene (pile on right).
CREDIT: INTERNATIONAL RICE RESEARCH INSTITUTE (IRRI)
Marker-assisted breeding is very focused, you don’t drag in genes that you don’t want, you can just drag in a very small region of a chromosome. And because the genetic fingerprint can be determined at the seedling stage, it saves a lot of time and labor that would normally be spent on submerging hundreds of plants.
Farmers have now been growing Sub1 varieties for several years. In 2017, more than 5 million farmers grew it. Sub1 rice is disproportionately benefiting the poorest farmers in the world, who often have the most flood-prone land. Compared with conventional rice varieties, farmers growing Sub1 rice are able to harvest three- to fivefold more grain after floods. The Intergovernmental Panel on Climate Change predicts that flooding will become more frequent and last longer as the climate changes.
These various breeding approaches underscore the difficulty in defining “genetically modified” crops. How do you define them?
The term “genetically modified” is scientifically meaningless, and so it’s not useful. The FDA does not use the term.
With Sub1 rice, for example, scientists can introduce the Sub1a gene with either genetic engineering or marker-assisted breeding. In each of these cases, the genetic region that’s introduced is smaller than the huge number of genes that you bring in with conventional breeding, in which you are mixing two genomes together.
Grafting is another kind of conventional breeding that mixes two genomes. There are a lot of grafted varieties on farms in California. The walnuts harvested in California are actually a graft of two different species where the rootstock is a different species than the top part of the plant. Then there are foods that we eat that have been developed through radiation and chemical mutagenesis, like grapefruit. Those approaches create many random uncharacterized changes in the genome and are not regulated. They can also be sold as “certified organic.”
What do you think most consumers mean when they say genetically modified organism or GMO?
I think some consumers are concerned only about plants engineered to contain genes from another species, like the bacterial Bt gene. It sounds a little strange to put bacterial genes into a plant, but it is important to consider the risks versus the benefits. Organic farmers spray Bt to prevent insect damage to their crops. It is safe to use. But spraying Bt is not always effective. In Bangladesh, for example, there is an insect that can destroy an entire eggplant crop and spraying doesn’t keep the insect from getting into the plant. And the Bt sprays are expensive and difficult to get. So Bangladeshi and Cornell scientists engineered eggplants with the bacterial gene so that the plants produce the Bt organic insecticide in the crop. And it’s been tremendously successful over the last five years, allowing farmers to reduce their insecticide sprays dramatically.
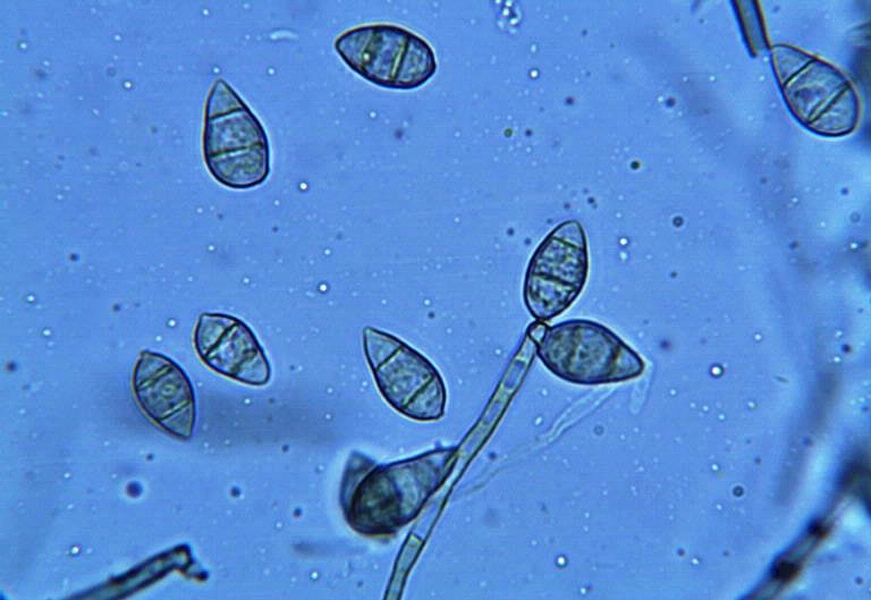
Among the challenges to feeding the world’s growing population is crops lost to disease. Developing rice strains that can resist infection by the extremely destructive rice blast fungus (spores shown) is an active area of research.
CREDIT: DONALD GROTH, USDA FOREST SERVICE
One reason that the FDA and many scientists don’t find the term “GMO” useful is because it means different things to different people. You can’t really compare an eggplant engineered for farmers in Bangladesh that has allowed them to reduce insecticide use to, say, the “Golden Rice” plants engineered to have higher amounts of provitamin A to help save the lives of children in developing countries, or herbicide-tolerant canola grown in developed countries. These are different traits, different crops, and different people benefit.
Why do you think there is so much distrust of modern genetic approaches?
I think part of the issue is that less than 2 percent of people in the US are farmers and are somewhat removed from food production. Many people aren’t familiar with the challenges faced by farmers and may not understand that Bt crops have massively reduced the use of insecticides in the US and globally. The World Health Organization estimates that 200,000 people die every year from misuse or overuse of insecticides, primarily in less developed countries.
The use of genetic technologies has become very politicized like several other issues in science — vaccines, climate change. The major scientific organizations have concluded that the climate is changing, that vaccines can save lives, and that genetically engineered crops are safe to eat and safe for the environment.
I think most of us know someone who has been very sick and we would do anything to help them. Often that means using a genetically engineered drug. Or maybe we know someone with diabetes who uses genetically engineered insulin. We accept that use of the technology, most consumers accept it, because they have some understanding of it in their own world. But I think very few Americans have seen a malnourished Bangladeshi kid, so it’s not in their world. It’s not that they aren’t compassionate, it’s that at some level they don’t understand or see it. They don’t really understand why farmers need genetically improved crops.
I think people understand with computer technology that there are different applications of that single technology. People wouldn’t say “computers are bad.” But somehow it gets confusing to people when it comes to agriculture, maybe because so many of us are so removed from actual farming.