It is a massive, unsolved problem. Chronic pain affects an estimated 50 million to 100 million Americans, disabling up to 20 million of them. And it’s more than just a noxious physical sensation — it affects attention, mood and sleep, even someone’s relationships and identity. Yet despite the enormous need for better treatments, there are few effective drugs to treat chronic pain beyond opioids, which can be dangerous and addictive. What’s the way ahead?
The search for better drugs is complicated by the fact that chronic pain takes different forms, and affects the brain as well as where the pain is “felt.” One strategy is to try to stop pain signals at the source: the nerves that sense damage or threats to the body.
For decades, neurologist and neuroscientist Stephen Waxman has been studying proteins called ion channels that allow these nerve cells to send their signals. Mutations in the genes carrying instructions for these channels give rise to rare pain disorders. These disorders and mutations are pointing the way to new medications for common pain conditions. Waxman, of the Yale School of Medicine and the VA Connecticut Healthcare System, recently coauthored an article about work toward this goal in the Annual Review of Neuroscience. He spoke with Knowable Magazine about the hunt for new pain treatments and the challenges of finding them.
This conversation has been edited for length and clarity.
What is pain, and where does it come from?
Pain is a complex phenomenon. Normal, or nociceptive, pain serves a very important protective and instructive role. If you put your finger near a fire, you immediately pull your finger away. We rapidly learn to avoid things that cause pain.
Nociception originates from receptors located in the peripheral nervous system: the nerves that run through our skin and organs. These receptors are activated by strong mechanical stimuli, noxious heat, noxious cold and noxious chemicals, and the signal is transmitted along nerves. These peripheral nerve fibers carry the pain signal to the spinal cord. There are other neurons within the spinal cord that relay the signal upward to the brain, where pain is recognized.
Now under some circumstances, pain is abnormal. There is a set of conditions, which fall under the term “neuropathic pain,” where these peripheral neurons fire spontaneously, even when there’s no threatening stimulus. It occurs in common disorders like diabetic neuropathy, shingles pain and a complication of cancer treatment called chemotherapy-induced peripheral neuropathy. In these disorders, peripheral pain-signaling neurons become hyperactive: They take on a life of their own and fire when they shouldn’t.
There’s also a set of pain disorders that we lump together as inflammatory pain. This pain is a result of damage to peripheral tissues, largely due to inflammation there. And again, this triggers inappropriate firing, which results in chronic pain.
How could understanding these nerve signals lead to new pain treatments?
Nerve cells communicate with each other by producing small electrical impulses. Those depend on the presence of a class of protein molecules called sodium channels. In a sense, you can think of them as tiny molecular batteries in the membrane of nerve cells. And these tiny molecular batteries produce tiny electrical currents that sum up and produce nerve impulses.
For many years, the scientific community talked about the sodium channel, as if there was only one type of sodium channel. As the molecular revolution rolled in, it became clear that there are several types of sodium channels, each encoded by a different gene. It turns out there are nine different sodium channels. We call them Nav1.1, 1.2, all the way through to Nav1.9 [Na for sodium and v for voltage-gated].
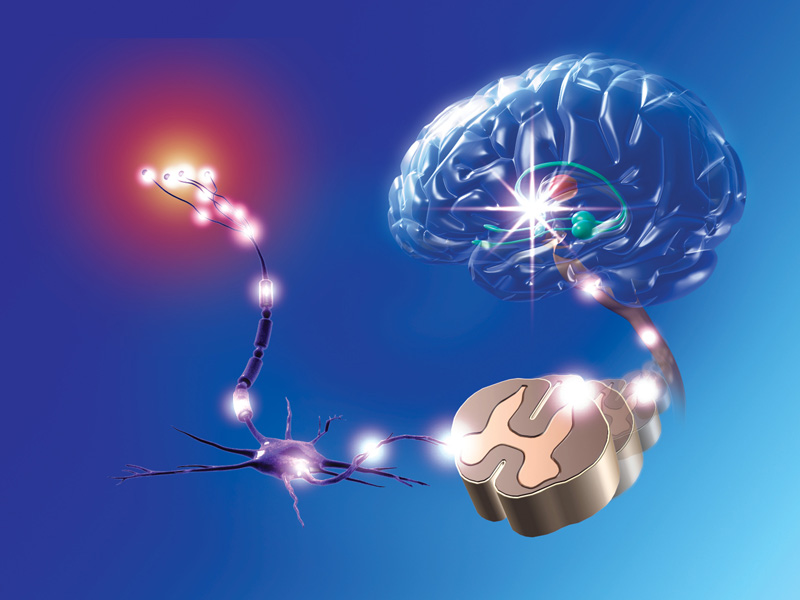
Painful stimuli are detected by peripheral sensory nerves called nociceptors (left), which send signals through the spinal cord to the brain, where they are recognized as pain.
CREDIT: MARC PHARES / SCIENCE SOURCE
So could those channels be blocked to relieve pain?
When we go to the dentist, we receive a local injection of novocaine or one of its derivatives. These are sodium channel blockers, and when you receive a local injection into or near the nerves innervating a tooth, there’s no pain. But if you took that same drug and put it in the form of a pill, you would also block sodium channels in the heart and brain, so you would get side effects in the central nervous system like double vision, loss of balance, sleepiness and confusion.
So early on, the question arose: Might there be a type of sodium channel that plays a key role in our peripheral nerves, particularly the pain-signaling peripheral nerves, but not in the brain? If those existed, you might be able to turn off pain signaling without central side effects. It turns out that there are three sodium channels that meet the criterion of being peripheral sodium channels; those are Nav1.7, Nav1.8 and Nav1.9.
Finding them was a huge focus of pain research, and now we know they exist. But having a target is just the beginning of the pathway to developing a new set of medications.
A lot of your work on these sodium channels involves people with rare diseases. How do they help our understanding?
In my laboratory, we first investigated people with a genetic disorder called inherited erythromelalgia. It’s also known as “man on fire syndrome,” because these people describe their pain as feeling as if hot lava had been poured into their body, or they’d been scorched with a flame thrower. The pain is triggered by mild warmth that most of us would interpret as almost imperceptible, or certainly not painful.
We discovered that individuals with inherited erythromelalgia all carry mutations in the same gene, the gene that encodes Nav1.7. You can think of the Nav1.7 channel as a volume knob on pain-signaling neurons, and in these individuals the volume knob is turned way up — their channels are overactive.
A few years after those families were found, the opposite mutations were found: families with loss-of-function mutations of Nav1.7. These people do not make functional Nav1.7 sodium channels, and they sustain painless bone fractures, painless childbirth, painless tooth extraction, painless burns. But these individuals don’t have any other apparent abnormalities of the central nervous system.
Scientists are starting to understand the root causes of certain inherited pain abnormalities. People with a rare genetic disease called inherited erythromelalgia feel pain much more intensely than normal because they carry a mutation that increases the activity of the sodium channel Nav1.7 in pain nerve cells. This causes the nerves to fire more readily. Others, with a congenital insensitivity to pain, carry a defective Nav1.7 sodium channel, so the pain nerves don’t fire. People with a third mutation, in the potassium channel Kv7.2, are resilient to pain because the mutation reduces the activity of pain nerves.
So that seems to suggest that, first of all, Nav1.7 is crucial for the sensation of pain?
That’s correct.
And secondly, that these sodium channels are found only or mainly in pain-sensing neurons?
In a broad-brush way, that’s correct. There may be small numbers of Nav1.7 channels in particular parts of the brain, but very importantly the individuals with the loss-of-function mutations of Nav1.7 don’t have any apparent neurologic abnormalities other than the inability to smell. The clinical studies that have been done on drugs that block Nav1.7 thus far have not yielded substantial side effects related to an effect on the brain.
Are there other rare disorders that might help here, too?
We occasionally encounter people with genetic mutations that should cause very, very extreme pain — like mutations in the gene for Nav1.7 that cause erythromelalgia — and who for some reason are resilient to developing that abnormal pain, although they still feel normal nociceptive pain. We’ve begun to study small numbers of such pain-resilient patients, and in those we have found other genetic variants in certain other genes that confer pain resilience. They do this by turning down the pain response and returning it to near normal.
Again, that may have implications for drug development, because by targeting those genes or the molecules produced by those genes, it may be possible to make an individual pain-resilient — to develop medications that will, in a sense, mimic pain resilience.
Can you give any hints about what types of molecules or what types of genes are making people pain-resilient?
We’re really excited about a gene that encodes a potassium ion channel called Kv7.2. This channel in a sense acts as a brake on neurons. It lowers their ability to produce nerve impulses and decreases the frequency of nerve impulse firing. In that respect, it acts the opposite to the Nav1.7 channel.
We’ve studied one individual in great detail. We know that because of her inherited erythromelalgia, she should have very severe pain — but her pain is very, very mild, and it’s because her Kv7.2 channels are overactive. So this opens up Kv7.2 as a potential target.
Why is it so hard to find new medications for pain?
When you’re trying to develop a new drug for cancer, you have biomarkers like blood counts, or you can do various types of scans and measure the size of the cancer. We don’t have that for pain — we ask patients to rate their pain on a scale from 0 to 10. So we don’t have objective measurements.
Another point is that animal studies have not produced drugs that work in people. Finally, people with pain can show a striking placebo response, which of course confounds measurements.
And these issues are superimposed on the general issues of how you develop a new medication. Having a molecule that works in the laboratory gets you only partway there. You have to engineer it into a deliverable form, and you have to make sure the side effect profile is acceptable and the drug is safe. You have to deal with things like dosage, all in a world where clinical trials are very costly, so you don’t get to do a lot of them.
When you put it all together, the challenge is immense. Despite that, I’m optimistic.
You’ve been doing this a long time, and there are a lot of challenges. What keeps you working on this problem?
Part of it is self-serving; it’s a lot of fun to be a biomedical scientist. But there’s also a component that serves others. On my wall I have a picture of two children with inherited erythromelalgia. We’ve learned so much from these children and their parents; they have been remarkably generous in sharing their DNA and their stories. The picture is to remind me that we’re part of a pipeline from the laboratory to society, and there are people depending on us.
On the hard days, when things don’t go perfectly well in the laboratory — and there are hard days — I look at that picture, I show it to my colleagues, and I say, “Look, the work we’re doing matters, it’s important for people who are depending on us.” And that’s something I find very motivating.