On a frosty day in early January, as Berlin’s trees and shrubs lie in deep dormancy, it’s hard to imagine it being much colder. But then I reach the Dahlem Seed Bank at the German city’s botanical gardens and botanist Elke Zippel guides me to a freezing chamber in the cellar, at a cool minus 11.2 degrees Fahrenheit, and the warmth rushes out of my body.
Around us are shelves of glass jars, jam-packed with vials of seeds. “There are millions of seeds,” says Zippel: minuscule black ones, rice-like ones and little brown pebble-like ones. The temperatures here have put them in a deep slumber intended to preserve them for the ages.
That’s for a good reason. The bank’s 12,000-plus collections include seeds of many rare or threatened European species — from the mountain arnica, a furry-leaved herb with healing properties that has lost much of its lowland habitat to intensive agriculture, to Gentianella uliginosa, a swamp-dwelling, purple-petaled flower that has become one of the continent’s rarest plants. All over the world, wild plants like these — vital members of ecosystems and unique products of eons of evolution — are losing ground. Over the past 250 years, around 570 species have gone extinct because of habitat destruction, invasive species and other threats.
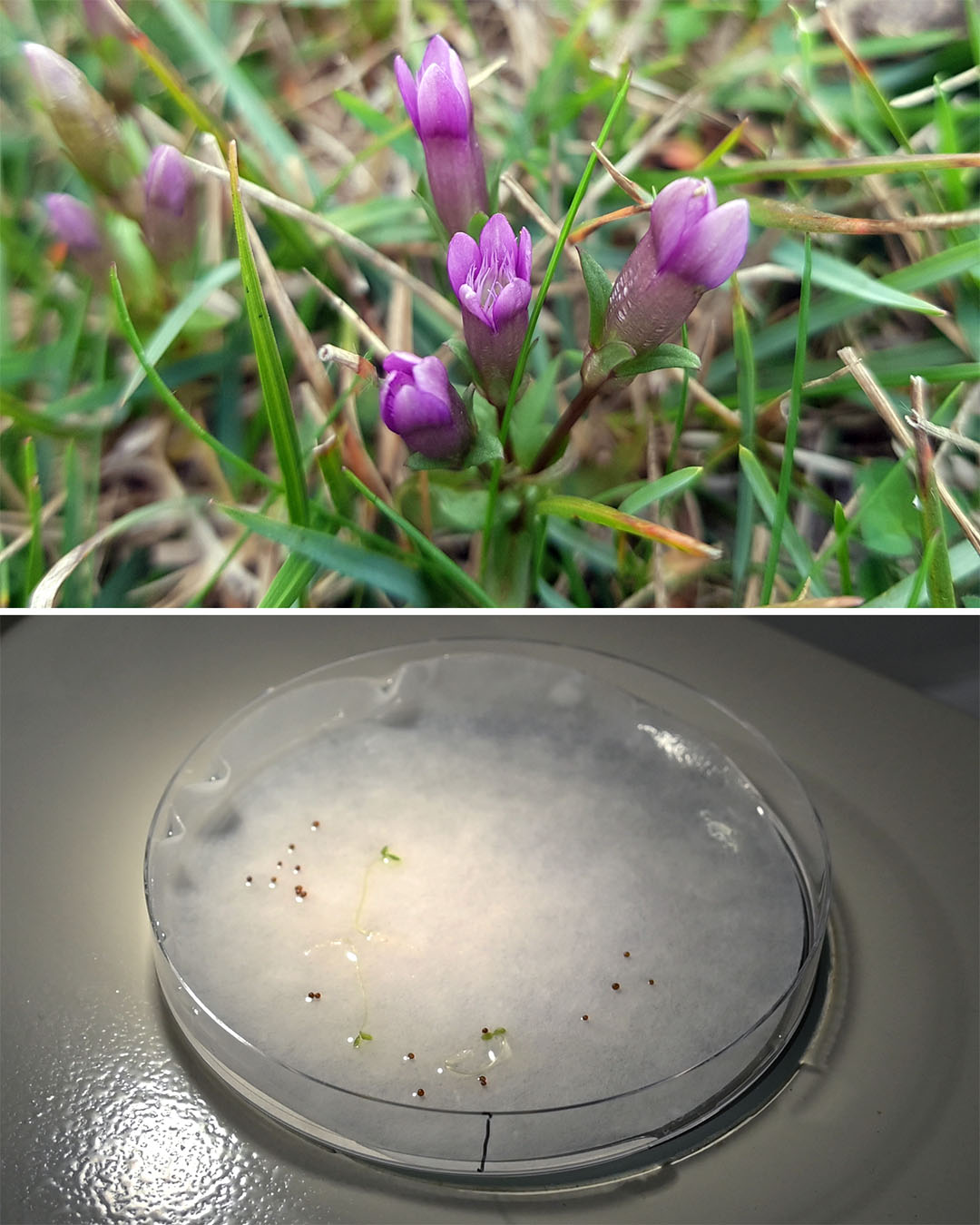
Botanists at the Dahlem Seed Bank in Berlin are running germination tests with seeds of the threatened swamp-dwelling plant Gentianella uliginosa, which has purple flowers (top panel). The goal is to see whether germination success can be improved in the presence of certain fungi (bottom panel).
CREDIT: ULLABRITTOLAND / iNATURALIST (TOP), KATARINA ZIMMER (BOTTOM)
Seeds offer a cost-effective way of preserving plants for future generations — hence intense efforts to bank the seeds of agricultural crops. There’s much less money available to preserve seeds of threatened and rare plants, but the Dahlem facility, like many seed banks around the world, is striving to do so, providing a last line of defense against extinctions and a reservoir of diversity to support dwindling populations.
But preserving seeds for future use is no simple task. Though seeds are living, breathing beings, many enter a deep metabolic slumber called dormancy, defiantly resisting germination until the time is right for them to sprout. It can be hard to wake them up. And while some seeds can long outlive people in this dozing state, many grow old and expire, even under ideal storage conditions.
Little by little, research is revealing what keeps seeds in deep sleep, how they age and die, and how to revive them when their time has come.
“Each species is its own little series of mysteries,” says botanist Wesley Knapp, the CEO of the California-based Center for Plant Conservation. “This is really frontline science, in many ways, and we’re trying to figure out what these very rare plants may need.”
The life and death of a seed
Seeds are essentially plant embryos, arising when pollen lands on a flower and fertilizes an egg cell; the embryos are often encased by energy-supplying tissues, a shell and sometimes fleshy fruit. To the amateur gardener, it may seem surprising that many are so little understood. After all, most cultivated and domesticated plant species — think petunias or tomatoes — have been bred to sprout quickly with soil, light and water. As long as the seeds are plonked in the ground before their expiration date, their metabolism swings into action, cells divide, a root shoots downward and a plant begins to grow.
But wild plants are meant to travel through time. For many of them, when their seeds dry out and fall dormant, their metabolism grinds to a halt and they resist germination for months, years or even decades. Plants do this as a bet-hedging strategy to stop their offspring from sprouting right away, in case conditions are bad for young seedlings — like in the middle of a dry summer, during a protracted drought or when there’s too much competing vegetation.
Scientists have learned that mother plants can take stock of environmental conditions and relay signals to developing seeds to prolong or shorten this dormancy. For example, when thale cress, a plant much studied in labs, experiences unusually chilly temperatures, it loads its fruit tissues with certain proteins; these proteins tweak the activity of genes in developing seeds so they stay dormant longer.
No seed lives forever. Although scientists report to have revived a 2,000-year-old date palm seed from an archaeological site in the Middle East, such cases are the exception. Knapp has struggled to revive century-old seeds from plants that are now extinct (see sidebar). And in an experiment that has been tracking seeds of 91 California native plant species since 1947, many seeds lost the ability to sprout in the first several decades, says Christina Walters, a seed biologist at the US Department of Agriculture who is curating the study.
But exactly why and how seeds age and die is something that scientists are still trying to understand. When seeds dry out — which most dormant seeds do — the cells inside transition from a fluid state to a solid, glassy state that protects them from degradation. Still, Walters suspects that seeds do accrue tiny physical and chemical changes over time; molecules within their cells undergo tiny rearrangements and become less able to power vital processes like energy production and protein-making.
The damage ultimately reaches a point of no return, says Walters, who is trying to identify the precise tipping point. “The seed — it’s alive, it’s alive, it’s alive, and then it dies,” she says.
Extending seed lives
Fortunately for threatened plants, there are ways of extending seed lives. At the Dahlem Seed Bank, Zippel takes me through a series of rooms for preparing seeds for freezing: one for drying them, another for cleaning them of fruit material and dust. Drying the seeds coaxes them into the desired glassy state and freezing them further winds down molecular movement. This can add years to seeds’ lives, from a few decades to hundreds of years, Zippel says.
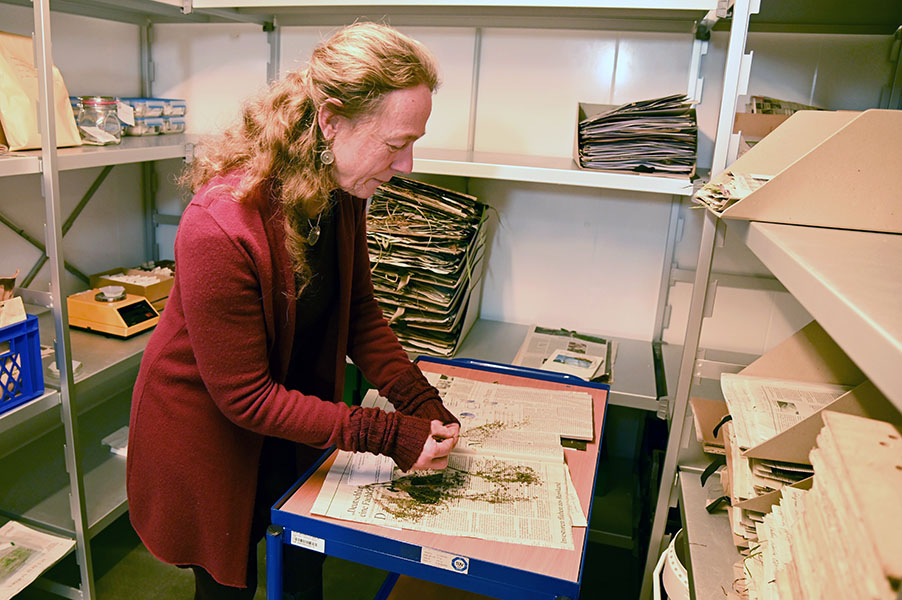
In the drying room at the Dahlem Seed Bank in Berlin, botanist Elke Zippel handles plants that were collected to preserve dried plant specimens as well as their seeds.
CREDIT: KATARINA ZIMMER
But not all seeds can be preserved this way — it works for “orthodox” seeds that can dry, which is key to safely freezing them. “Recalcitrant” seeds like acorns and chestnuts contain a lot of water and don’t tolerate drying. Freezing these seeds under normal conditions causes the water inside them to expand, crushing and killing the cells.
This is where cryopreservation comes in, explains seed- and cryobiologist Manuela Nagel of the Leibniz Institute of Plant Genetics and Crop Plant Research, who coauthored an article on cryopreservation in the 2024 Annual Review of Plant Biology. The most common method involves very rapidly freezing plant tissue to ultra-low temperatures — usually by placing it in liquid nitrogen below minus 238 degrees Fahrenheit — so ice crystals don’t have time to form. But each species seems to need its own cryopreservation protocol. “Finding out what the plant tolerates can be challenging and take years,” Nagel says.
At the Sussex-based Millennium Seed Bank of the Royal Botanic Gardens, Kew, for instance, seed biologist Louise Colville is working to cryopreserve acorns of British oak species. It’s part of Kew’s effort to establish a cryobank for plants that can’t be preserved in traditional seed banks. Since even cryopreservation requires tissues to have as little water content as possible, she and her colleagues usually excise the oak embryos inside acorns, partially dry them and rapidly freeze them.
But it has taken much trial and error to get this to work. The embryo tissues brown as soon as they’re exposed to air, and they seem to be extremely sensitive to drying and nitrogen exposure. “Even with a really great protocol, a success rate of 40 percent survival is considered good,” Colville says.
Colville and her colleagues are also trying to cryopreserve seagrass seeds to help with efforts to restore grassy marine habitats. They hope to preserve pollen, spores, shoot tips and dormant buds as well, from plants that don’t produce seeds. “It really does open up the possibilities for conserving a much wider range of species,” she says.
But cryopreservation, just like seed banking, doesn’t provide infinite longevity. And the only way to know when a seed collection has given up the ghost is to do regular germination tests, which are time-consuming and risk depleting precious seed collections when working with rare species.
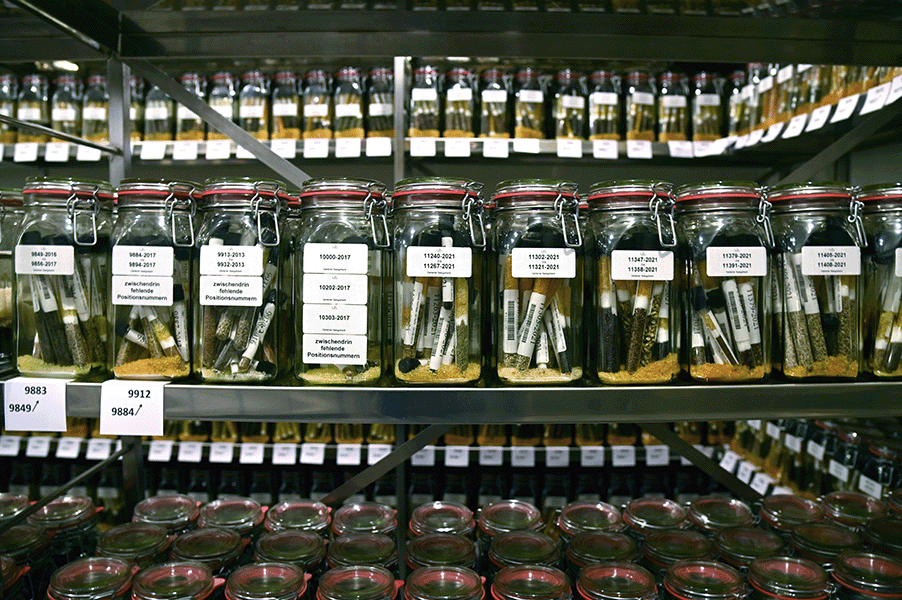
In a frigid cellar room at the Dahlem Seed Bank, kept at minus 11.2 Fahrenheit, seeds from a large variety of European species are being preserved for the ages. Many of them are from threatened and rare plants.
CREDIT: KATARINA ZIMMER
That’s why Walters is exploring other ways to measure seed viability and predict life expectancy. She has learned, for example, that the small snippets of genetic material called RNA turn to fragments over time — and this could be measured by extracting RNA molecules from seeds and sorting them by size. This serves as a kind of clock that reflects a seed’s age.
The oil molecules in seeds also break apart and create impurities that affect how the oils crystallize when they’re cooled. These changes become apparent when analyzing the amount of energy absorbed by seeds as they’re warmed up past the melting point of their fats, which can be done without destroying the seeds.
If researchers could catch viability declines early enough by such methods, it would make it easier to know when to grow plants from seeds while they can still germinate, and collect their seeds for storage, keeping the collection going.
Stubborn seeds
Even if seeds are viable, waking them up can be a challenge in and of itself. In fact, the very definition of dormancy is that seeds won’t sprout even when given soil, water and light. “If a seed doesn’t want to germinate because it’s not the right time,” Zippel says, “it will not germinate.”
Some seeds, like those of many plants in the celery and carrot family, need time for their embryos to fully mature after leaving the mother plant, sprouting only weeks or months later. Beans often have a very tough seed coat that prevents water from entering, and only when this is broken — by something such as wildfire heat, the acid of a bird’s stomach, a scalpel or sandpaper — can water enter and the seed awaken.
But the most common form of seed dormancy is also the toughest to break. Seeds in such states take up water, which revs up their metabolism. But then the metabolism quickly winds back down and they go to back to sleep, explains seed physiologist Dennis Brandt of the University of Münster in Germany. Researchers have worked out that such seeds resist germination by maintaining a certain balance of two plant hormones — abscisic acid and gibberellin — inside the seed.
There’s a tension between these two hormones. Abscisic acid kick-starts a cascade of biochemical reactions that repress cell division and growth, while gibberellin has the opposite effect. Until the balance tips in favor of gibberellin, the seed will continue to snooze.
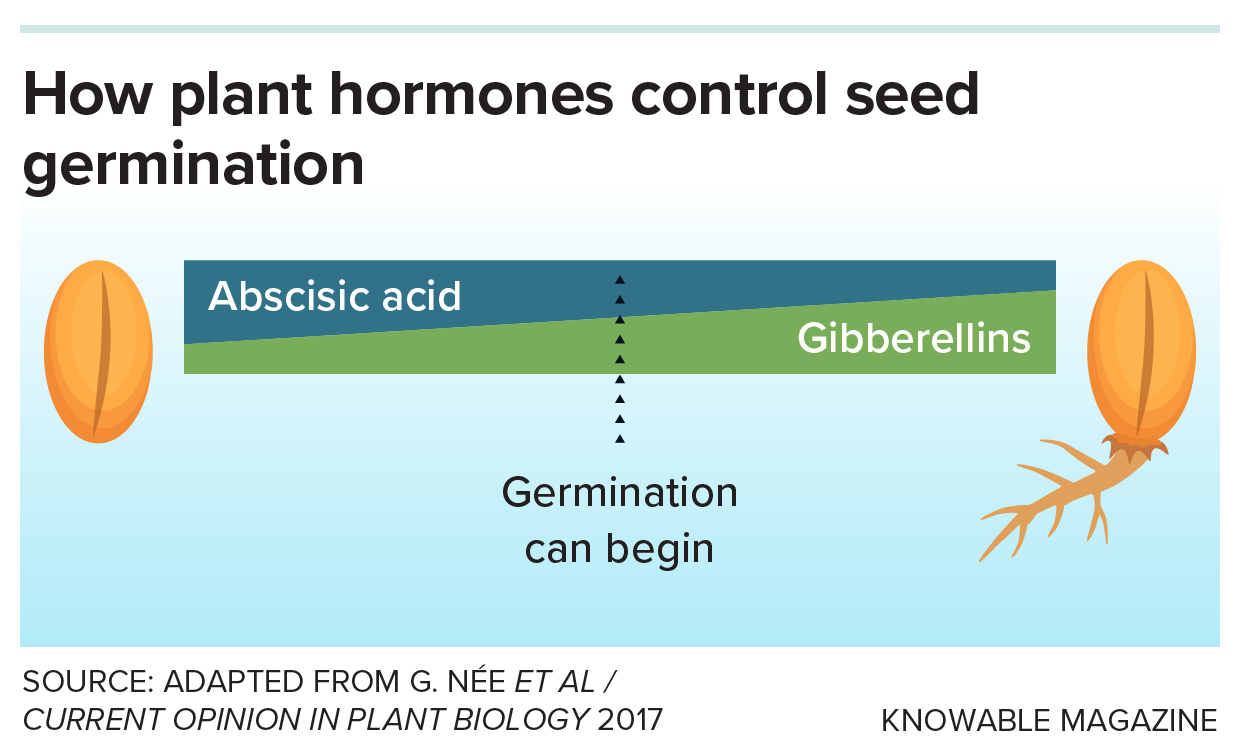
In some seeds, the switch from dormancy to germination is controlled by a balance between two classes of plant hormones: abscisic acid, which maintains dormancy by repressing cell division, and gibberellins, which trigger germination by fueling growth. Seeds have evolved elaborate mechanisms to tip the balance in favor of gibberellins in response to certain triggers such as light or temperature changes. Only then can seeds start to grow.
And that’s just part of the puzzle. More recently, scientists have realized that a protein called “Delay of Germination 1” — or DOG1 — also plays an important role in prolonging dormancy. It does so by acting on other proteins and genes, with the details still to be figured out. Many seeds require both abscisic acid and DOG1 to maintain dormancy, Brandt says.
What exactly are the seeds waiting for? The answer — ideal conditions for germination — has led to the evolution of elegant triggers. Some seeds have light-detecting molecules called phytochromes under their seed coats that prompt gibberellin production in the presence of red light — the only wavelengths that trickle through a forest canopy, signaling a shady, protected spot to sprout. Other seeds need darkness, to ensure germination in deep soil layers. And some need a period of cold — signaling the end of winter and the imminent arrival of spring. That cold, in some plants, triggers changes in the amounts of DOG1 and the destruction of abscisic acid, so that a seed’s metabolism fully springs into action.
Interestingly, different populations of the same species can have stronger or weaker dormancies that dovetail with their needs in different environments, Brandt says. And differences can also occur within the offspring of a single plant, ensuring that seeds don’t all germinate at the same time. This could be beneficial when there’s a sudden spell of spring warmth followed by a cold wave, he adds, “possibly killing the early-germinating seedlings but likely not the ones which did not germinate yet.”
And there’s much more to learn: Scientists have focused mostly on well-studied laboratory models like thale cress and are only scratching the surface of dormancy-breaking processes in many species, Brandt says, especially the rare and exotic ones that are most in need of conserving.
Breaking dormancy
While some species have simple and obvious germination triggers, other species have evolved unique — and sometimes very elaborate — solutions.
“A lot of things in the lily family need a warm and wet stratification before cold and wet, and then when you shift them back to a warm situation, they’ll finally germinate,” says plant ecologist Michael Kunz of the North Carolina Botanical Garden.
The globe bladderpod, a yellow-flowered mustard losing its habitat to roads and agriculture, has germination triggers that vary greatly across populations and even within the same mother plants. At the Missouri Botanical Garden, ecologist Matthew Albrecht has found that seeds respond variably to different combinations of light, temperature and increased levels of nitrates around them — signaling that vegetation around the seed has decayed, providing nutrients and space for young seedlings.
“These species that have this bet-hedging seed dormancy strategy — those are some of the trickiest species to deal with,” Albrecht says.
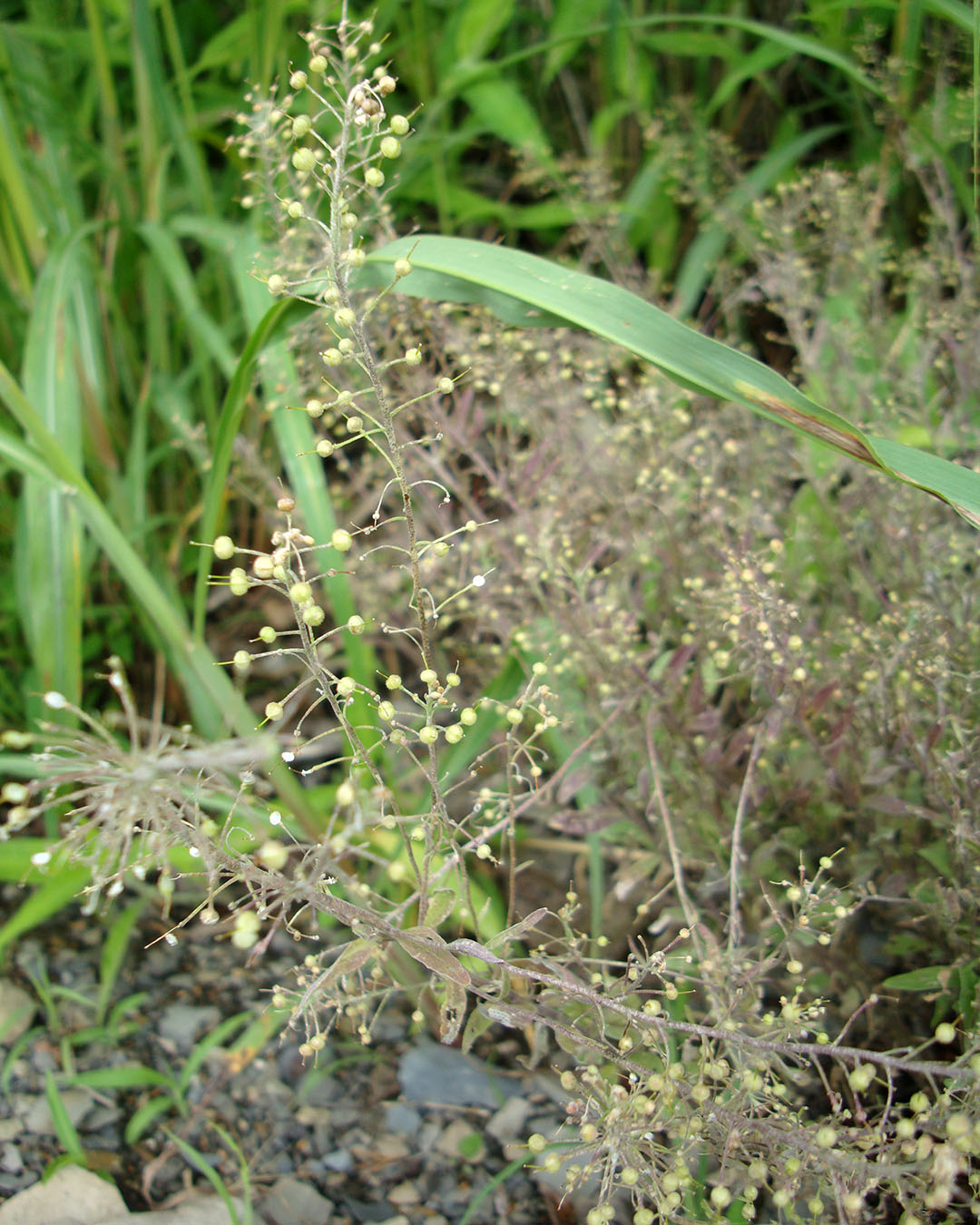
The seeds of the globe bladderpod, a plant in the mustard family, can have remarkably different germination triggers. Seeds from different populations, or even from the same mother plant, respond to different combinations of light, temperature and chemical changes in the soil. Working out how to germinate these seeds may help to preserve the species, which is losing its habitat to road developments and agricultural expansion.
CREDIT: MATTHEW ALBRECHT
And there are seeds, such as from wild beet and certain plants in the umbel family, that some scientists say they can’t get to sprout no matter what they do. Researchers estimate they only know surely how to propagate less than ten percent of the 1,200 rare species stored in the banks of the California Plant Rescue, a cross-organization effort that collects and preserves seeds from around the state. “If all we had to do was collect the seeds, put them in storage and then never deal with them again, that is one thing,” says Naomi Fraga, who directs conservation programs at the California Botanic Garden, one of the rescue’s member organizations. But, she adds, “if we don’t know how to grow them, then what is the point?”
Some triggers have taken decades to work out. In the mid-1990s, restoration ecologist and botanist Kingsley Dixon of the University of Western Australia was struggling to grow seedlings of dozens of species needed for a mining site restoration project. Because those plants evolved to sprout after bushfires — when flames have cleared out competitors and produced nutrient-rich soils — Dixon wondered if exposing them to heat would break their dormancy. But this had little effect.
After getting a clue from a conference presentation by South African researchers, he finally realized the trigger might be smoke. And sure enough, when he blew smoke from burning leaves into a germination tent or poured smoke-infused water over the soil, little sprouts emerged from seeds of the purple-petaled fringed lily.
Eleven years later, Dixon’s team finally identified, among 4,000 chemical compounds, the specific molecules in smoke that trigger germination in hundreds of Australian species and many from elsewhere. The team named them karrikins after a word for fire used by the Aboriginal Noongar people. The chemicals are probably produced at the steam-producing front of a fire, and seep with the water through the soil into the seeds, which “find it irresistible,” Dixon says. Some research suggests that karrikins spur gibberellin production, kick-starting growth.
Back in 1998, Dixon revived a species feared to be extinct: the Corrigin grevillea. The plant had last been spotted decades earlier, growing by a highway in the town of Corrigin in western Australia’s wheat belt, where most land has been cleared for agriculture. Scientists marked the spot and crossed their fingers that dormant seeds still lay in the soil. When Dixon and his colleagues arrived, they erected tents around the area and pumped in smoke — and, finally, saw seedlings emerging. “It was extraordinary,” Dixon recalls.
Ever since, Australian botanists have been wielding smoke to wake up dormant seeds. Within the past three years, they’ve revived a near-extinct cousin of the Corrigin grevillea, the Foote’s grevillea, and a small, threatened herb known as Sowerbaea multicaulis.
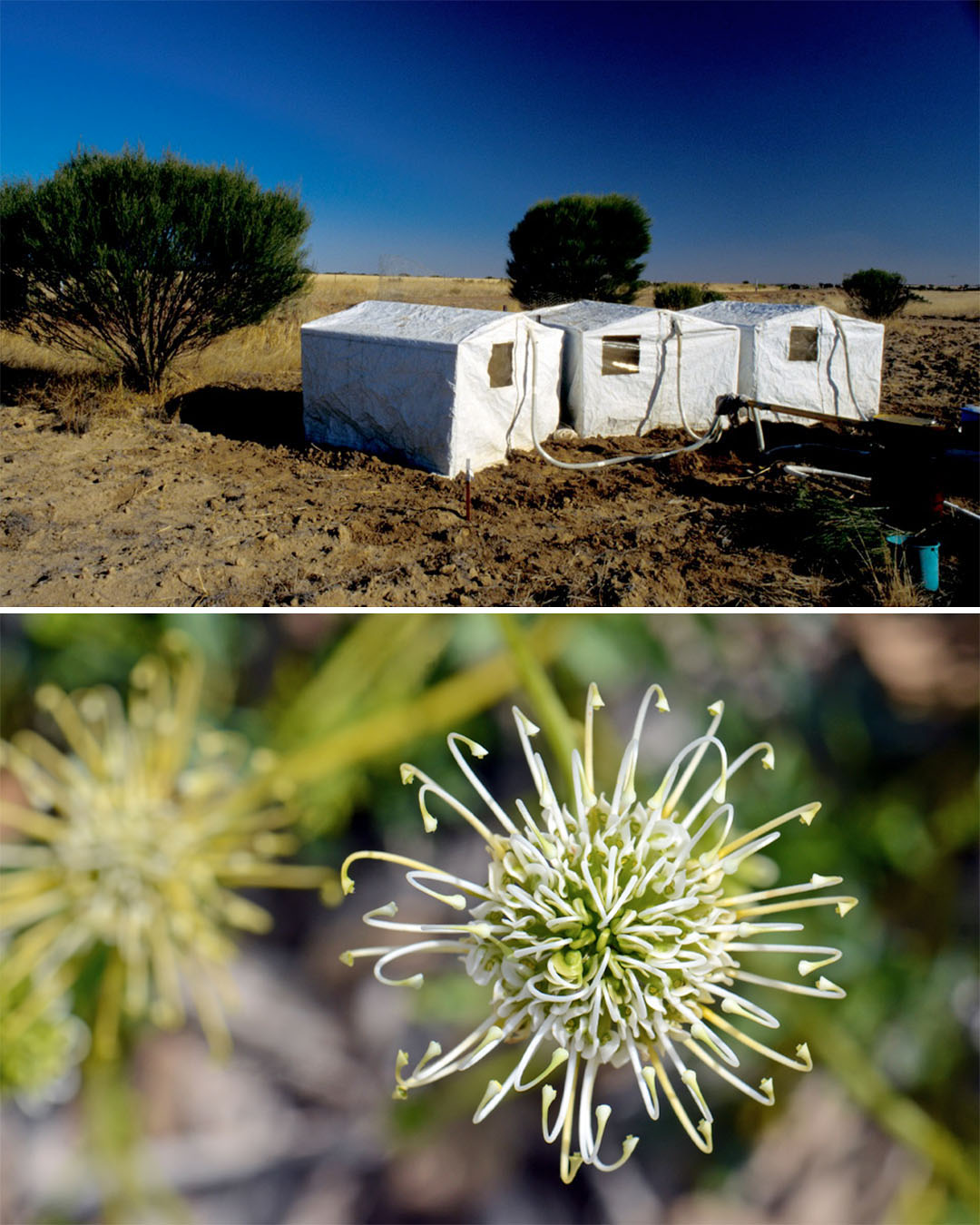
The Australian plant Grevillea scapigera, known as the Corrigin grevillea (bottom panel) was once thought to be extinct. But at the spot where it was last seen, scientists were able to reawaken seeds that lay dormant in the soil by exposing them to smoke, which they blasted into tents erected around the site (top panel).
CREDIT: KINGSLEY DIXON (TOP), ISTOCK.COM / KARENHBLACK (BOTTOM)
There are still mystery plants in the Australian desert and wildflower lands, including some native blueberries that Dixon has been trying to germinate since 1982; he suspects they may require a very particular sequence of environmental cues.
From light to smoke, there’s a great bet-hedging benefit to ecosystems in having such a diversity of dormancy-breaking processes across species and even within them, Dixon says. “It’s like the Las Vegas of ecology: They’re playing every table in the room.”
From seed to ecosystem
Tricky though it is, germinating young seedlings is just the first step in rebuilding self-sustaining wild plant populations, from identifying fungi they need to grow with to protecting them against challenges like drought. But there are success stories.
At a military base in North Carolina, Kunz and his colleagues have used preserved seeds to restore populations of the Georgia false indigo, the sandhills milkvetch and the sandhills lily.
In several natural areas managed by Tennessee government agencies, Albrecht’s team has made progress in restoring a purple-flowered legume known as Pyne’s ground plum, much of whose original habitat has been covered by Nashville developments.
And on Germany’s Baltic Sea coast, Zippel’s colleagues successfully restored populations of the mountain arnica. “Now the population is growing by itself,” she says.
These are small victories, but they matter in a world where threats to plants continue to mount. As Zippel stashes seeds away in her freezing basement, as biologists further unlock the secrets of dormancy, and as botanists work to revive and raise young seedlings for return to the wild, these scientists hope they can give all threatened plants an essential lifeline.
Editor’s note: This article was updated on April 28, 2025, to clarify that the Millennium Seed Bank of the Royal Botanic Gardens, Kew, is based at the Sussex site of the organization, not the London site. It was updated on April 30, 2025, to correct the credit for the seed photos shown in the collage. The photos are courtesy of M. Cubre, © BO Berlin, not the Dahlem Seed Bank.