Animals use physics? Let us count the ways
Cats twist and snakes slide, exploiting and negotiating physical laws. Scientists are figuring out how.
Support sound science and smart stories
Help us make scientific knowledge accessible to all
Donate today
Isaac Newton would never have discovered the laws of motion had he studied only cats.
Suppose you hold a cat, stomach up, and drop it from a second-story window. If a cat is simply a mechanical system that obeys Newton’s rules of matter in motion, it should land on its back. (OK, there’s some technicalities — like this should be done in a vacuum, but ignore that for now.) Instead, most cats usually avoid injury by twisting themselves on the way down to land on their feet.
Most people are not mystified by this trick — everybody has seen videos attesting to cats’ acrobatic prowess. But for more than a century, scientists have wondered about the physics of how cats do it. Clearly, the mathematical theorem analyzing the falling cat as a mechanical system fails for live cats, as Nobel laureate Frank Wilczek points out in a recent paper.
“This theorem is not relevant to real biological cats,” writes Wilczek, a theoretical physicist at MIT. They are not closed mechanical systems, and can “consume stored energy … empowering mechanical motion.”
Nevertheless, the laws of physics do apply to cats — as well as every other kind of animal, from insects to elephants. Biology does not avoid physics; it embraces it. From friction on microscopic scales to fluid dynamics in water and air, animals exploit physical laws to run or swim or fly. Every other aspect of animal behavior, from breathing to building shelters, depends in some way on the restrictions imposed, and opportunities permitted, by physics.
“Living organisms are … systems whose actions are constrained by physics across multiple length scales and timescales,” Jennifer Rieser and coauthors write in the current issue of the Annual Review of Condensed Matter Physics.
While the field of animal behavior physics is still in its infancy, substantial progress has been made in explaining individual behaviors, along with how those behaviors are shaped via interactions with other individuals and the environment. Apart from discovering more about how animals perform their diverse repertoire of skills, such research may also lead to new physics knowledge gained by scrutinizing animal abilities that scientists don’t yet understand.
Critters in motion
Physics applies to animals in action over a wide range of spatial scales. At the smallest end of the range, attractive forces between nearby atoms facilitate the ability of geckos and some insects to climb up walls or even walk on ceilings. On a slightly larger scale, textures and structures provide adhesion for other biological gymnastics. In bird feathers, for instance, tiny hooks and barbs act like Velcro, holding feathers in position to enhance lift when flying, Rieser and colleagues report.
Biological textures also aid movement by facilitating friction between animal parts and surfaces. Scales on California king snakes possess textures that allow rapid forward sliding, but increase friction to retard backward or sideways motion. Some sidewinding snakes have apparently evolved different textures that reduce friction in the direction of motion, recent research suggests.
Small-scale structures are also important for animals’ interaction with water. For many animals, microstructures make the body “superhydrophobic” — capable of blocking the penetration of water. “In wet climates, water droplet shedding can be essential in animals, like flying birds and insects, where weight and stability are crucially important,” note Rieser, of Emory University, and coauthors Chantal Nguyen, Orit Peleg and Calvin Riiska.
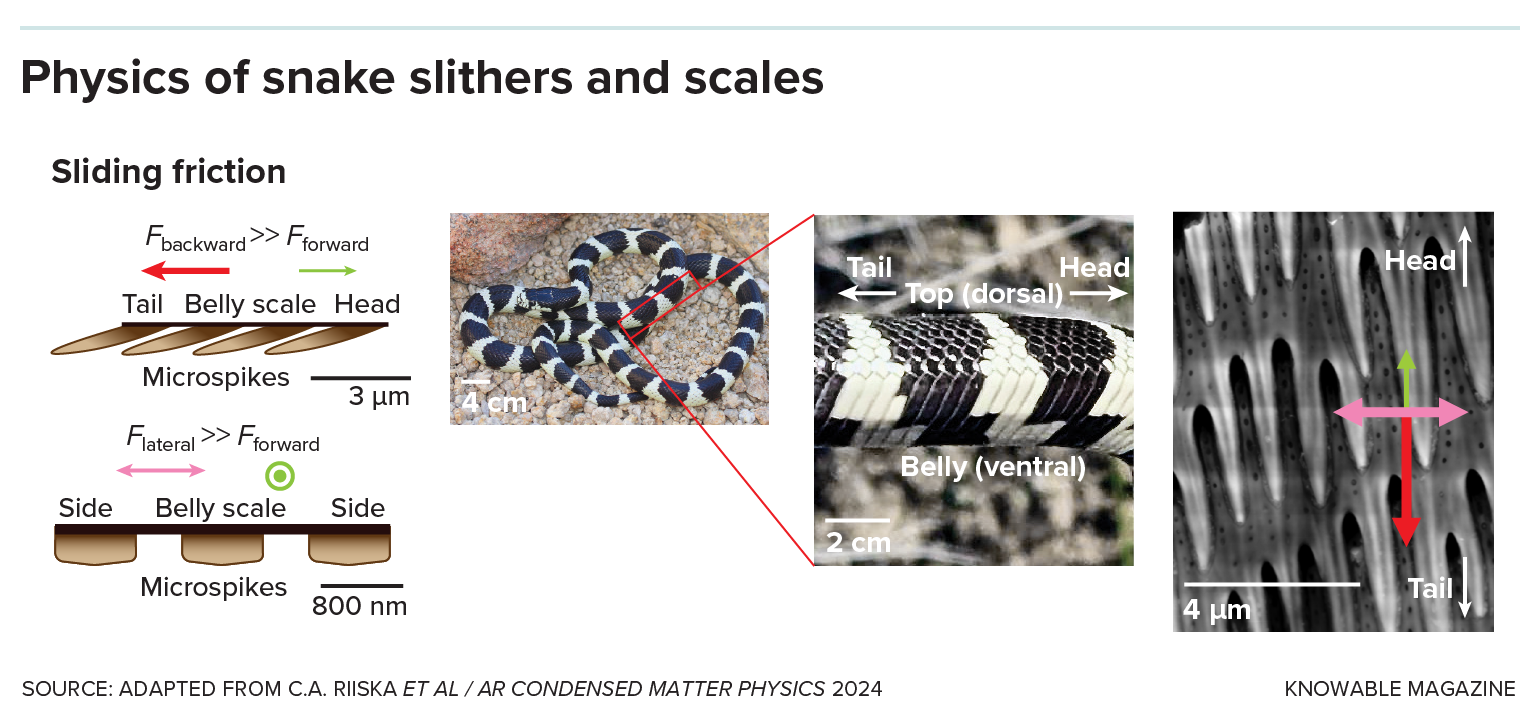
The physics of friction determines how different shapes and structures of snakeskin scales influence the ways that snakes slither. Microspikes angle away from the head of a California king snake, creating friction that counters any backward slide but smooths forward movement.
Water-blocking surfaces also help animals keep their skins clean. “This self-cleansing mechanism … can be important to help protect the animal from dangers like skin-borne parasites and other infections,” the Annual Review authors explain. And in some cases, removing foreign material from an animal’s surface may be necessary to preserve the surface properties that enhance camouflage.
In fact, the physics of how light interacts with an animal’s surface is relevant for many other behaviors. Bright colors and iridescence in birds, butterflies and some other insects depend on how layers of different microstructures are combined. Such colors contribute to courting and can influence the ability to avoid predators.
At larger scales, physics still underlies even the simplest animal movements, which require a complex coordination of electrical and chemical signaling within the body and between body and brain. And for successful motion, internal physics must mesh with the physical properties of the environment. Moving through a fluid, for instance, is governed not only by the body but also by the properties of the liquid.
In water, swimming animals employ different moving strategies depending on various factors, including the shape of their body. Fish with slim bodies, for instance, basically propel themselves by side-to-side motion of body and tail. Fish of many other body shapes generate motion by moving their fins.
Physics methods for describing these strategies cannot easily account for factors like turbulence and whirlpools. Calculating expected behavior in such situations can exceed available computing power. So scientists have turned to actual experiments. One such study provided clues to a peculiar ability of trout; water flowing past a cylinder induced a vortex that allowed even a dead trout to swim upstream.
Animal assembly
Evolution has provided animals with movement skills adapted to the existing environment without any need for an instruction manual. But altering the environment to an animal’s benefit requires more sophisticated physics savvy. From ants and wasps to badgers and beavers, various animals have learned how to construct nests, shelters and other structures for protection from environmental threats.
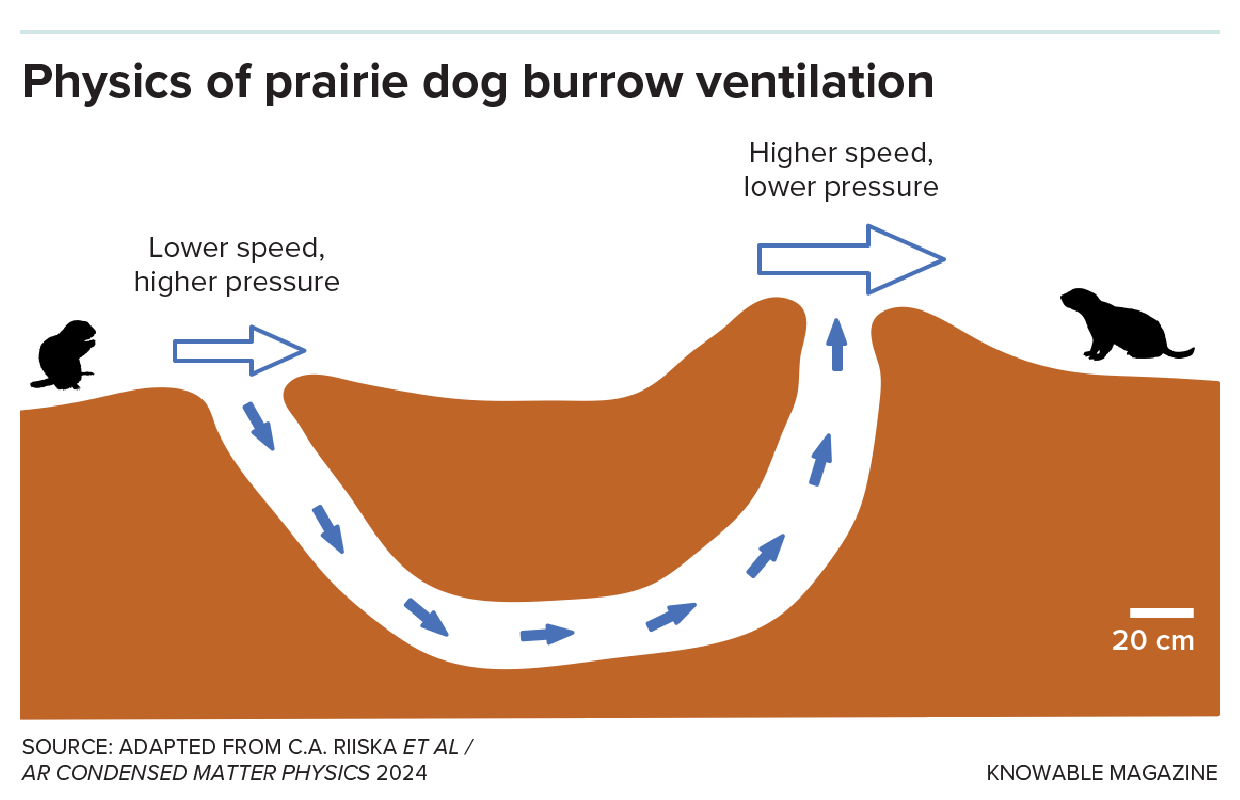
Prairie dogs dig burrows with multiple entrances at different elevations, an architecture that relies on the laws of physics to create airflow through the chamber and provide proper ventilation.
Bird nests, for instance, must combine sticks and leaves and dirt and grass into a structure of dependable stability and mechanical integrity. Birds apparently know that flexible sticks or twigs provide better stability than rigid rods; physics experiments have shown that the bending of the more flexible materials enables frictional forces that help hold the nest together. Rieser and colleagues suspect that applying more bird knowledge about assembling nest components could help scientists design novel metamaterials for various purposes.
Animal structures must also obey the physics necessary to control temperature, humidity and ventilation within a comfortable range. “Without sufficient air exchange, for instance, animals would suffocate,” Rieser and colleagues write.
Prairie dogs, for example, build extensive burrows with multiple openings. Those openings should differ in elevation, physics analysis shows, to provide proper ventilation (via pressure differences that induce airflow). Field studies show that prairie dog engineers have figured that out for themselves — just as cats figured out how to twist and change body shape when falling.
No doubt animals have many other tricks that physicists themselves can’t yet fully explain, which is why the field of animal behavior physics is so fertile.
“Further investigations of the many facets of animal behaviors from a physics perspective,” Rieser and colleagues write, “will … aid in the discovery of new physical laws for behavior that nature has figured out but that we have yet to uncover or fully understand.”
10.1146/knowable-071024-1
TAKE A DEEPER DIVE | Explore Related Scholarly Articles